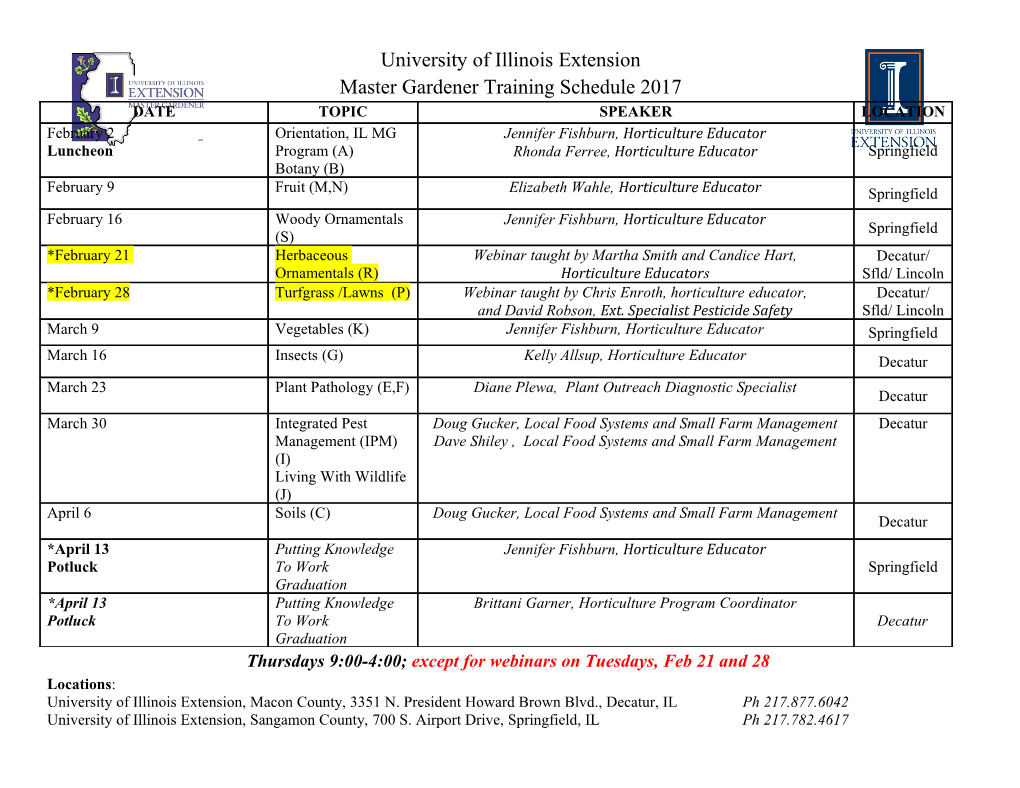
VISUALIZATION OF THE RIBBON SYNAPSE USING RIBEYE A-MCHERRY FUSION PROTEIN by Megan C. West Submitted in partial fulfillment of the requirements For the degree of Master of Science Thesis Advisor: Dr. Brian M. McDermott Department of Biology CASE WESTERN RESERVE UNIVERSITY August, 2011 CASE WESTERN RESERVE UNIVERSITY SCHOOL OF GRADUATE STUDIES We hereby approve the thesis/dissertation of Megan C. West candidate for the Master’s of Science degree *. (signed) Michael F. Benard (chair of the committee) Heather Broihier Brian M. McDermott Roy E. Ritzmann (date) June 10, 2011 *We also certify that written approval has been obtained for any proprietary material contained therein. TABLE OF CONTENTS LIST OF FIGURES …………………………………………………………………………………………………………….4 ABSTRACT………………………………………………………………………………………………………………………5 INTRODUCTION………………………………………………………………………………………………………………6 Chemical and Electrical Synapses………………………………………………………………………..6 Conventional Chemical Presynaptic Active Zones……………………………………………….7 The Ribbon Synapse……………………………………………………………………………………………9 Ribeye protein………………………………………………………………………………………………….12 Hair Cells…………………………………………………………………………………………………………..13 Zebrafish as a Model Organism…………………………………………………………………………15 Zebrafish Inner Ear Development and Lateral Line Development……………………..17 Modes of Investigating the Ribbon Synapse……………………………………………………..19 MATERIALS AND METHODS………………………………………………………………………………………….20 RESULTS……………………………………………………………………………………………………………………….29 DISCUSSION………………………………………………………………………………………………………………….45 REFERENCES…………………………………………………………………………………………………………………51 3 LIST OF FIGURES FIGURE 1………………………………………………………………………………………………………………………..7 FIGURE 2………………………………………………………………………………………………………………………..8 FIGURE 3………………………………………………………………………………………………………………………10 FIGURE 4………………………………………………………………………………………………………………………14 FIGURE 5………………………………………………………………………………………………………………………17 FIGURE 6………………………………………………………………………………………………………………………18 FIGURE 7………………………………………………………………………………………………………………………24 FIGURE 8………………………………………………………………………………………………………………………26 FIGURE 9………………………………………………………………………………………………………………………30 FIGURE 10…………………………………………………………………………………………………………………….33 FIGURE 11…………………………………………………………………………………………………………………….36 FIGURE 12…………………………………………………………………………………………………………………….37 FIGURE 13…………………………………………………………………………………………………………………….38 FIGURE 14…………………………………………………………………………………………………………………….39 FIGURE 15…………………………………………………………………………………………………………………….43 FIGURE 16…………………………………………………………………………………………………………………….44 TABLE 1………………………………………………………………………………………………………………………..21 4 Visualization of the ribbon synapse using Ribeye a-mCherry fusion protein Abstract by MEGAN C. WEST Specialized presynaptic matrices known as ribbon synapses are found in sensory cells of the visual, auditory, and vestibular systems of vertebrates. Hair cells of the auditory system and the vestibular system rely on ribbon synapses to transmit signals to the brain. Due to the lack of tools to visualize the ribbon synapse, studying the ribbon has been challenging. We designed the construct, Tg(pvalb3b:ribeye a-mCherry), which allows for visualization of ribbon synapses in the hair cells of live or fixed zebrafish. We show that the Ribeye a-mCherry fusion protein expressed from this construct localizes to appropriate areas using immunolabeling and live animal imaging. These results demonstrate that Tg(pvalb3b:ribeye a-mCherry) is an effective tool to label ribbons. We utilize this tool to characterize mutant zebrafish and to investigate ribbon dynamics. Our results show that Ribeye a-mCherry has the ability to be used in mutant zebrafish and in fluorescent recovery after photobleaching experiments. 5 Introduction Chemical and Electrical Synapses. The nervous system is a complicated network comprised of an extremely large number of cells. The main signaling cell in the nervous system is known as a neuron. In order for the nervous system to operate as a functional unit, there must be communication between neurons. The synapse is the site of communication between neurons and can be either electrical or chemical. Most synapses in vertebrates are chemical synapses (Kandel and Siegelbaum, 2000). Electrical synapses use an ion current to transmit information from one cell to another; however, chemical synapses use chemical transmitters to convey information. Synapses are comprised of a presynaptic structure and a postsynaptic structure. The morphology of these structures depends on the type of synapse. At electrical synapses, continuity between the presynaptic and postsynaptic cells is established by gap junctions channels found in each cell. In chemical synapses there is no contact between the two cells and the space between them is known as the synaptic cleft (Figure 1). The presynaptic cell of a chemical synapse houses neurotransmitter-filled vesicles. These chemicals are exocytosed into the synaptic cleft and eventually bind to receptors found on the postsynaptic cell. 6 Figure 1. Schematic of a chemical synapse. The presynaptic cell is filled with vesicles (blue circles) containing neurotransmitter (black dots). These vesicles dock at the plasma membrane, where they undergo vesicle 'priming'. When an electrical impulse (wavy white line) arrives in the presynaptic cell the vesicles fuse to the active zone plasma membrane and neurotransmitter is released into the synaptic cleft. Receptors on the postsynaptic cell detect the neurotransmitter, and trigger a postsynaptic electrical response. Reprinted with permission from Macmillan Publishers Ltd: Nature, Dobrunz and Garner, 2002. www.nature.com Conventional Chemical Presynaptic Active Zones. Chemical synapses contain active zones which are areas of the plasma membrane specialized for the release of neurotransmitter. These areas are regulated through voltage gated Ca2+channels which, when open, cause a depolarization of the cell and allows synaptic vesicles to fuse with the presynaptic membrane and release neurotransmitter (Figure 1). The active zone plasma membrane is characterized by an electron-dense meshwork associated with the plasma membrane, the presence of synaptic vesicles, and its alignment opposite of neurotransmitter receptors on the postsynaptic cell. There are two main components of the active zone, the active zone plasma membrane and the cytomatrix at the active zone (CAZ). 7 The CAZ is a complex structure which extends ~ 50 nm into the cytoplasm (Gray, 1963; Pfenninger et al., 1972; Phillips et al., 2001). Many proteins found at the CAZ have been identified and can be grouped into six categories: (1) proteins involved in synaptic vesicle fusion, including syntaxin and SNAP-25; (2) cytoskeletal proteins, such as actin, tubulin, and myosin; (3) scaffolding proteins, such as CASK; (4) voltage gated calcium channels; (5) cell adhesion molecules, including neurexins and cadherins and (6) CAZ-specific proteins known as UNC13/Munc13 proteins, Rab3-interacting molecule (RIMs), ELKS (ERC/CAST), and Bassoon and Piccolo/aczonin (Fejtova and Gundelfinger, 2006). These molecules work together to form the scaffold of the CAZ as well as organize the synaptic vesicle cycle (Figure 2). The CAZ is believed to have the important functions of positioning the neurotransmitter release site opposite the postsynaptic receptors and supplying the active zone with synaptic vesicles (Zhai et al., 2001). Figure 2. Molecular organization of the CAZ. This schematic shows several molecules working together to anchor the CAZ and assist in the synaptic vesicle cycling. Synaptic vesicles (SV) are depicted as circles with neurotransmitter (black) present inside. Reprinted with permission from Springer, Schoch and Gundelfinger, 2006. 8 Many studies have been conducted to understand the functions of CAZ-specific proteins. The UNC13/Munc13 proteins have been shown to be involved in synaptic vesicle ‘priming’, which prepares the vesicles for fusion with the plasma membrane (Brose et al., 2000). It has also been suggested that these proteins may function in the regulation of presynaptic short-term plasticity (Rosenmund et al., 2002). RIMs are scaffolding proteins known to interact with many of the proteins found at the CAZ. Studies have shown that RIMs also function in priming and short-term plasticity as well as long-term plasticity (Calakos et al., 2004). ELKS proteins are believed to have important interactions with CAZ specific proteins. These interactions may be required to localize RIMs to the active zone and a disruption of the interaction between ELKS and Bassoon results in reduced neurotransmitter release (Ohtsuka et al., 2002; Takao-Rikitsu et al., 2004). These three protein families, UNC13/Munc13,RIMs, and ELKS, are conserved in C. elegans, Drosophila, and vertebrates (Fejtova and Gundelfinger, 2006) In contrast, Bassoon and Piccolo are not believed to be conserved in C. elegans and Drosophila but are found in vertebrates; these two proteins are the largest active-zone specific proteins (Fejtova and Gundelfinger, 2006). Piccolo and Bassoon are proposed to be involved in synaptic vesicle clustering (Mukherjee et al., 2010) and Bassoon has been shown to play an important part in the assembly and function of the CAZ (Chen et al., 2011). The Ribbon Synapse. Ribbon synapses are specialized CAZs found in sensory cells in the auditory, visual, and vestibular systems. The ribbon synapse is characterized by the appearance of an electron dense structure known as the ribbon or dense body, which is 9 surrounded by a halo of vesicles. These vesicles are tethered to the ribbon through
Details
-
File Typepdf
-
Upload Time-
-
Content LanguagesEnglish
-
Upload UserAnonymous/Not logged-in
-
File Pages59 Page
-
File Size-