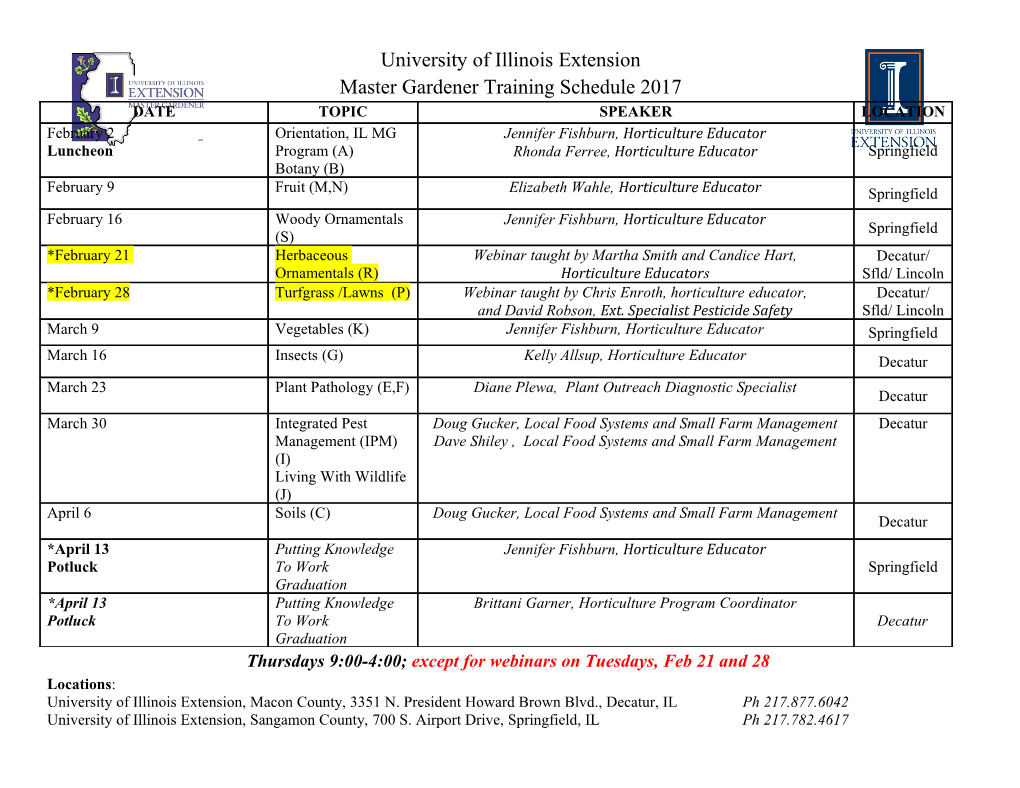
15 January 2002 Optics Communications 201 (2002) 391–397 www.elsevier.com/locate/optcom Frequency noise characterisation of narrow linewidth diode lasers L.D. Turner, K.P. Weber, C.J. Hawthorn, R.E. Scholten* School of Physics, University of Melbourne, Parkville, Vic. 3010, Australia Received 4 October 2001; received in revised form 4 October 2001; accepted 15 November 2001 Abstract We examine several approaches to laser frequency noise measurement in the frequency and time domains. Com- monly employed methods such as optical frequency discrimination and the Allan variance are found to be complex, expensive, time-consuming, or incomplete. We describe a practical method of demodulating a laser beat note to measure a frequency noise spectrum, using a phase-locked loop frequency discriminator based on a single low-cost integrated circuit. This method measures the frequency noise spectrum of a laser directly and in detail and is insensitive to intensity fluctuations. The advantages of this scheme are demonstrated through measurement of the frequency noise spectrum for two external cavity diode lasers (ECDL), clearly distinguishing several common noise sources. These are isolated and removed, reducing the individual laser rms linewidth from 2 MHz to 450 kHz. The spectrum is used to calculate the Allan variance, which shows almost none of the important information. Ó 2002 Elsevier Science B.V. All rights reserved. PACS: 42.55.-f; 42.55.Px; 42.60.Mi; 42.60.-v Keywords: Diode lasers; Frequency noise; Frequency discrimination 1. Introduction tem, the identification and removal of frequency noise sources is crucial to narrowing the linewidth. Narrow linewidth and highly stable lasers are Accurate measurement of the laser frequency critically important in fields as diverse as optical stability is also often required as an experimental communications, laser cooling and atomic parameter. frequency standards. When designing a laser sys- External cavity diode lasers (ECDLs) are a common example of such narrow linewidth lasers [1]. ECDLs use semiconductor diode lasers in an external cavity with dispersive feedback, often from a diffraction grating. The linewidth is greatly * Corresponding author. Tel.: +61-3-8344-5457; fax: +61-3- 9347-4783. reduced with respect to the diode alone, and the E-mail address: [email protected] (R.E. laser can be tuned through the broad gain curve of Scholten). the diode. 0030-4018/02/$ - see front matter Ó 2002 Elsevier Science B.V. All rights reserved. PII: S0030-4018(01)01689-3 392 L.D. Turner et al. / Optics Communications 201 (2002) 391–397 ECDLs exhibit varying forms of frequency 2.2. Radio frequency discrimination noise from environmental, fundamental, and ar- tificial sources. Environmental noise includes 50 Laser frequency noise can also be measured by or 60 Hz power-line-induced noise, and acousti- heterodyning two lasers, for example by superim- cally coupled noise, in particular at frequencies posing the two beams on a fast photodiode (PD) to corresponding to mechanical resonances. Funda- produce a radio frequency (rf) beat note at the dif- mental noise is typically dominated by white ference frequency. Frequency fluctuations apparent phase noise at high frequencies and flicker in the beat note are the combination of the indi- frequency noise (also known as 1=f noise or pink vidual frequency fluctuations of the lasers. A single noise) at low frequencies [2]. The flicker laser can also be superimposed with itself via a fiber frequency noise is often the dominant component delay loop [7], but such self-homodyne systems are of the linewidth, and so it is common practice to ill-suited to measuring laser noise at low frequen- stabilise the laser to an external frequency refer- cies, since impracticably long delays are required. ence, for example a Fabry–Perot etalon or sub- The heterodyne method is very commonly used Doppler atomic resonance. The feedback systems to obtain a measure of the linewidth of a laser. The usually rely on dithering the laser frequency [3] or beat note is analysed with an rf spectrum analyser the reference frequency [4], but in both cases it is and the width of the spectral peak at the beat common to find significant noise at the dither frequency, averaged over some measurement time, frequency, thus introducing an artificial source of is taken as the combined laser linewidth. Unfor- frequency noise. tunately this provides very little information on the composition, and hence sources, of the fre- quency noise [2]. 2. Measurement of laser frequency noise Laser frequency noise may be measured directly 3. Analysis of radio frequency spectra at the optical frequency, or by heterodyning to a reference laser. Frequency noise analysis of the beat signal can provide this information, either in the frequency domain or in the time domain. The beat note sig- 2.1. Optical frequency discrimination nal produced by lasers with frequency difference m0 has voltage Direct optical measurements use an optical V t V t sin 2pm / t ; 1 frequency discriminator to convert frequency ð Þ¼ 0ð Þ ½ 0 þ ð Þ ð Þ modulation to intensity modulation. Common where V t describes amplitude fluctuations of the 0ð Þ examples are transmission at the side of a Fabry– two lasers and / t is the difference of the indi- Perot etalon fringe [5], or the side of an atomic vidual phases. Theð instantaneousÞ beat frequency is absorption resonance [6], either Doppler-broad- 1 d/ t ened or sub-Doppler. These can be convenient and m t m0 ð Þ m0 Dm t 2 low cost, but high frequency resolution, signal to ð Þ¼ þ 2p dt ¼ þ ð ÞðÞ noise ratio, and stability are more difficult to with frequency fluctuations Dm t m0. achieve. In addition, such optical frequency dis- ð Þ criminators typically do not distinguish between 3.1. Time domain frequency analysis frequency and intensity fluctuations. This lack of distinction between the two types of fluctuations is The time domain parameters are variances of particularly important when analysing the fre- multiple measurements of the instantaneous quency noise of diode lasers, because fluctuations frequency m t each of period s. The standard in the injection current affect both frequency and measure is theð Þ Allan variance, the zero dead-time output power. two-sample deviation over a given time period s: L.D. Turner et al. / Optics Communications 201 (2002) 391–397 393 discriminator which outputs a voltage propor- 2 1 2 r s ms t ms t s ; 3 tional to Dm t , with minimal dependence on am- ð Þ¼2 D½ ð Þ À ð þ Þ E ð Þ ð Þ plitude fluctuations V0 t . The power spectral where the brackets denote time averaging. The density of this voltage, forð Þ example acquired with measurement of anhi Allan variance requires two an audio frequency spectrum analyser, is SDm. frequency counters, a pulse sequencer, and a Previous experiments using discriminators have computer with data acquisition system to calculate required complex rf electronics [12] and suffered the variance at each time interval [8]. from poor spectral resolution [13]. We describe a simple frequency discriminator and its application 3.2. Frequency domain analysis to ECDLs, and demonstrate the increased infor- mation over the rf beat spectrum alone and over The fundamental parameter in the frequency Allan variance measurements. domain is the frequency noise power spectral 2 We use a single chip phase-locked loop (Philips density, measured in Hz =Hz, given by SA568), which consists of a local voltage con- 1 trolled oscillator phase-locked to the input signal SDm f 2 Dm t Dm t s exp i2pf s ds; with a phase detector and feedback loop. The ð Þ¼ Z0 h ð Þ ð þ Þi ðÀ Þ feedback voltage depends on the frequency of the 4 ð Þ input signal. Unlike previously employed dis- where f is termed the Fourier frequency. The root criminators, this frequency discriminator operates mean square (rms) linewidth Dmrms is then across a broad range of beat note frequencies from 40 to 110 MHz, tracks frequency deviations of up 2 1 to 10 MHz, and is insensitive to amplitude mod- Dmrms SDm f df : 5 ¼ Z0 ð Þ ð Þ ulation (20 dB rejection). The beat note linewidth measured on an rf spec- Fig. 1 is a schematic of our apparatus. The trum analyser is not simply related to the rms single frequency output beams from two ECDL linewidth. If the frequency noise spectrum is a delta [14] are superimposed on a New Focus 1621 function, the rf beat spectrum will be approxi- photodetector. The beat signal was amplified by 32dB with a wideband MMIC (Agilent MSA- mately rectangular with width of L 2p2Dmrms. If the rf beat spectrum is Gaussian, the¼ fullffiffiffi width at 0886) and fed directly into the SA568. Our circuit half maximum (FWHM) will be 2:35Dmrms [9]. The Allan variance may be determined from the frequency noise spectrum SDm by the integral [10] 4 2 1 sin pf s r s 2 SDm f ð 2 Þ df 6 ð Þ¼ Z0 ð Þ pf s ð Þ ð Þ but in general the frequency noise spectrum cannot be determined from the Allan variance [11]. The frequency noise spectrum is therefore preferable to the Allan variance. 4. Experiment Fig. 1. Two external cavity diode lasers (ECDLs), locked to saturated absorption resonances, were superimposed with a 4.1. Phase-locked loop frequency discriminator non-polarising beamsplitter (NPS) onto a fast photodiode (PD). The heterodyne signal was pre-amplified and analysed with a frequency discriminator (Philips SA568) and the fre- The frequency noise spectrum may be recovered quency spectrum acquired with any one of the audio frequency directly from the beat signal with a frequency spectrum analysers shown.
Details
-
File Typepdf
-
Upload Time-
-
Content LanguagesEnglish
-
Upload UserAnonymous/Not logged-in
-
File Pages7 Page
-
File Size-