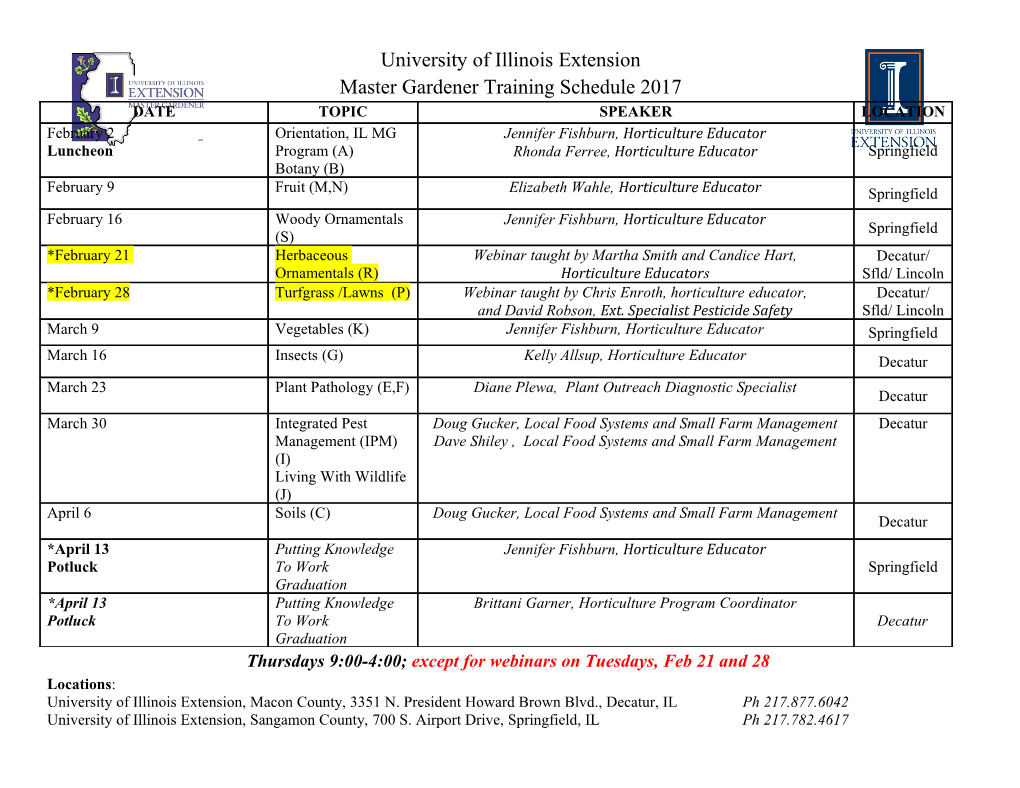
Structure and two-metal mechanism of a eukaryal nick-sealing RNA ligase Mihaela-Carmen Unciuleaca, Yehuda Goldgurb, and Stewart Shumana,1 aMolecular Biology Program, Sloan-Kettering Institute, New York, NY 10065; and bStructural Biology Program, Sloan-Kettering Institute, New York, NY 10065 Edited by James M. Berger, The Johns Hopkins University School of Medicine, Baltimore, MD, and approved October 1, 2015 (received for review August 19, 2015) ATP-dependent RNA ligases are agents of RNA repair that join 3′-OH via clade-specific acquisitions of flanking domain modules (7). and 5′-PO4 RNA ends. Naegleria gruberi RNA ligase (NgrRnl) exemplifies DNA ligases and RNA capping enzymes have a shared core a family of RNA nick-sealing enzymes found in bacteria, viruses, and domain structure in which a conserved oligonucleotide-binding eukarya. Crystal structures of NgrRnl at three discrete steps along the (OB)–fold domain is linked to the C terminus of the NTase reaction pathway—covalent ligase-(lysyl-Nζ)–AMP•Mn2+ intermediate; domain (8–14). The scheme depicted in Fig. 1 posits that: (i)ATP- 2+ 2+ ligase•ATP•(Mn )2 Michaelis complex; and ligase•Mn complex— dependent DNA ligases evolved before divergence of bacteria, ar- + highlight a two-metal mechanism of nucleotidyl transfer, whereby (i) chaea, and eukarya; (ii)NAD-dependent ligases evolved in bac- an enzyme-bound “catalytic” metal coordination complex lowers the teria by acquisition of a signature N-terminal module that confers + pKa of the lysine nucleophile and stabilizes the transition state of the NAD specificity (15, 16); and (iii) capping enzymes evolved ATP α phosphate; and (ii) a second metal coordination complex bridges uniquely in eukarya, via innovations within the NTase and OB the β-andγ-phosphates. The NgrRnl N domain is a distinctively embel- domain that impart GTP specificity and recognition of diphosphate- lished oligonucleotide-binding (OB) fold that engages the γ-phosphate terminated RNA ends. and associated metal complex and orients the pyrophosphate leaving In contrast, ATP-dependent RNA ligases appear to have evolved group for in-line catalysis with stereochemical inversion at the AMP many times, and independently, by NTase fusions to structurally phosphate. The unique domain architecture of NgrRnl fortifies the diverse C-terminal domain modules (Fig. 1). There are presently theme that RNA ligases have evolved many times, and independently, four structurally characterized RNA ligase families, exemplified by: by fusions of a shared nucleotidyltransferase domain to structurally (i) bacteriophage T4 RNA ligase 1 (Rnl1 family) (17); (ii) T4 RNA diverse flanking modules. The mechanistic insights to lysine adenylyla- ligase 2 (Rnl2 family) (18); (iii) Pyrococccus abyssi RNA ligase tion gained from the NgrRnl structures are likely to apply broadly to (Rnl3 family) (19); and (iv) Clostridium thermocellum RNA ligase the covalent nucleotidyltransferase superfamily of RNA ligases, DNA (Rnl4 family) (20, 21). The C-domain folds of these four RNA ligases, and RNA capping enzymes. ligases are unrelated to one another and to the OB domains of DNA ligases or capping enzymes. The physiology and biochemistry RNA repair | covalent nucleotidyltransferase | lysyl-AMP of the Rnl families suggest a division of labor in RNA repair, whereby Rnl1 and Rnl4 are tailored to seal single-strand breaks in iochemically diverse RNA repair systems rely on RNA ligases the loop of RNA stem-loops (22, 23), whereas Rnl2 is designed to ′ ′ Bto maintain or manipulate RNA structure in response to seal 3 -OH/5 -PO4 nicks in duplex RNAs and RNA:DNA hybrids purposeful RNA breakage events (1–5). RNA breaks destined (24). The specificity of T4 Rnl1 for tRNA repair is conferred by its for repair are inflicted by sequence-specific or structure-specific C domain (22). T4 Rnl2 depends on its C domain for binding and endoribonucleases during physiological RNA processing (e.g., sealing a duplex RNA nick (7, 25). Our hypothesis is that there are tRNA splicing; kinetoplast mRNA editing) and under conditions more RNA ligase families to be discovered and that new flavors of of cellular stress (e.g., virus infection, starvation, unfolded protein response). RNA cleavage can occur either: (i) by a transesterification Significance mechanism (generally metal-independent) that yields 2′,3′-cyclic-PO4 and 5′-OH ends; or (ii) via a hydrolytic mechanism (typically metal- Polynucleotide ligases are an ancient superfamily of nucleic acid ′ ′ dependent) that leaves 3′-OH and 5′-PO4 ends. RNA repair enzymes repair enzymes that join 3 -OH and 5 -PO4 DNA or RNA ends. + – capable of sealing 2′,3′-cyclic-PO4/5′-OHbreaksor3′-OH/5′-PO4 Ligases react with ATP or NAD to form a covalent enzyme breaks are distributed widely in all phylogenetic domains of life. adenylate intermediate in which AMP is linked via a P–N bond ATP-dependent RNA ligases join 3′-OH and 5′-PO4 RNA to a lysine side-chain. This paper reports crystal structures of a termini via a series of three nucleotidyl transfer steps similar to eukaryal ATP-dependent RNA ligase (Naegleria gruberi RNA those of DNA ligases (6). In step 1, RNA ligase reacts with ATP ligase, NgrRnl) that illuminate the stereochemistry and two-metal to form a covalent ligase-(lysyl-Nζ)–AMP intermediate plus pyro- catalytic mechanism of the lysine adenylylation reaction. A sig- phosphate. In step 2, AMP is transferred from ligase-adenylate to nature N-terminal domain of NgrRnl binds the ATP γ-phosphate and orients the pyrophosphate leaving group apical to the lysine the 5′-PO4 RNA end to form an RNA–adenylate intermediate, AppRNA. In step 3, ligase catalyzes attack by an RNA 3′-OH on nucleophile. NgrRnl is the founder of a distinct RNA ligase clade, the RNA–adenylate to seal the two ends via a phosphodiester bond with homologs in diverse bacterial, viral, and eukaryal proteomes. and release AMP. Author contributions: M.-C.U. and S.S. designed research; M.-C.U. and Y.G. performed The autoadenylylation reaction of RNA ligases is performed research; M.-C.U., Y.G., and S.S. analyzed data; and M.-C.U., Y.G., and S.S. wrote by a nucleotidyltransferase (NTase) domain that is conserved in the paper. + ATP-dependent and NAD -dependent DNA ligases and GTP- The authors declare no conflict of interest. dependent mRNA capping enzymes (6, 7). The NTase domain This article is a PNAS Direct Submission. includes six peptide motifs (I, Ia, III, IIIa, IV, and V) that form Data deposition: The atomic coordinates have been deposited in the Protein Data Bank, the nucleotide-binding pocket (Fig. S1). Motif I contains the www.pdb.org (PDB ID codes 5COT, 5COU, and 5COV). lysine that becomes covalently attached to the NMP. It is thought 1To whom correspondence should be addressed. Email: [email protected]. that modern RNA and DNA ligases and RNA capping enzymes This article contains supporting information online at www.pnas.org/lookup/suppl/doi:10. evolved from an ancestral stand-alone ATP-using NTase domain 1073/pnas.1516536112/-/DCSupplemental. 13868–13873 | PNAS | November 10, 2015 | vol. 112 | no. 45 www.pnas.org/cgi/doi/10.1073/pnas.1516536112 Downloaded by guest on September 25, 2021 amoeba,” which causes a devastating infection, primary amoebic meningoencephalitis.) We have purified and characterized recombi- nant NgrRnl, the exemplary eukaryal Rnl5 ligase (29). Here we report atomic structures of NgrRnl, captured at three discrete steps along the lysine–adenylylation reaction pathway. The struc- tures suggest a two-metal mechanism of nucleotidyl transfer, in which a catalytic metal coordination complex lowers the pKa of the lysine nucleophile and stabilizes the transition state of the ATP α-phosphate, and a second metal coordination complex bridges the ATP β-andγ-phosphates. The NgrRnl N domain is a uniquely embellished OB-fold that binds the ATP γ-phosphate and asso- ciated metal complex and orients the pyrophosphate leaving group for in-line catalysis. Results Overview of the NgrRnl Structure. Crystallization of native and SeMet-substituted NgrRnl and structure determination of the native enzyme at 1.7 Å resolution are described in Methods and summarized in Table S1. A stereoview of the NgrRnl tertiary structure is shown in Fig. 2A. It is composed of 21 β-strands, seven α-helices, and two 310 helices, which are displayed over the amino acid sequence in Fig. 2B. NgrRnl consists of two domains. As expected, the C-terminal module (amino acids 123–339) is a classic NTase domain. A DALI search (33) of the PDB recovered the NTase domain of T4 Rnl2 as the top “hit,” with a z-score of Fig. 1. Scheme of ligase evolution. RNA ligases, DNA ligases, and mRNA cap- α ping enzymes comprise a superfamily of covalent nucleotidyltransferases that 18.4 and 2.4 Å rmsd at 186 C positions (Table S2). The aligned act via enzyme-(lysyl-Nζ)–NMP intermediates. They are thought to descend from tertiary structures of the NgrRnl and T4 Rnl2 NTase domains are an ancestral ATP-using NTase domain (turquois oval) by fusions to structurally shown in Fig. S1A. The primary structure alignment in Fig. S1B diverse flanking domain modules (depicted in various shapes and colors), as discussed in the text. RNA ligases will inform the biochemistry, biology, and evolutionary history of RNA repair. Deinococcus radiodurans RNA ligase (DraRnl) and Naegleria gruberi RNA ligase (NgrRnl) represent a recently appreciated, though structurally uncharacterized, Rnl5 family of ATP-dependent RNA ligase (26–29). DraRnl and NgrRnl are template-directed RNA ligases capable of sealing nicked duplexes in which the 3′-OH strand is RNA. DraRnl and NgrRnl can join a RNAOH endtoa 5′-pRNA or 5′-pDNAstrand,butareunabletojoinwhenthe3′-OH strand is DNA. In this respect, they are similar to T4 Rnl2. However, unlike members of the four known RNA ligase families, DraRnl and BIOCHEMISTRY NgrRnl lack a C-terminal appendage to their NTase domain.
Details
-
File Typepdf
-
Upload Time-
-
Content LanguagesEnglish
-
Upload UserAnonymous/Not logged-in
-
File Pages6 Page
-
File Size-