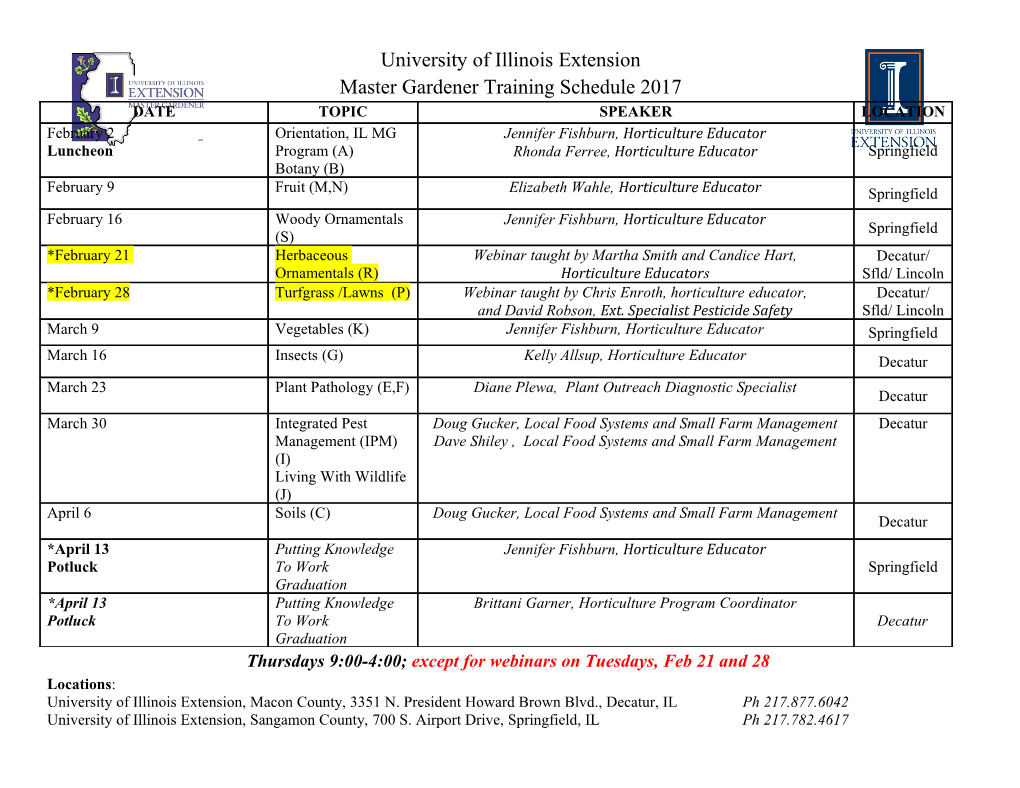
Dissertations and Theses Fall 2014 Investigation of Pulse Detonation Engine Flow Conditions for Turbomachinery Integration Luis Estefano Ferrer-Vidal Espa˜na-Heredia Embry-Riddle Aeronautical University - Daytona Beach Follow this and additional works at: https://commons.erau.edu/edt Part of the Propulsion and Power Commons Scholarly Commons Citation Espa˜na-Heredia, Luis Estefano Ferrer-Vidal, "Investigation of Pulse Detonation Engine Flow Conditions for Turbomachinery Integration" (2014). Dissertations and Theses. 158. https://commons.erau.edu/edt/158 This Thesis - Open Access is brought to you for free and open access by Scholarly Commons. It has been accepted for inclusion in Dissertations and Theses by an authorized administrator of Scholarly Commons. For more information, please contact [email protected]. Investigation of Pulse Detonation Engine Flow Conditions for Turbomachinery Integration by Luis Estefano Ferrer-Vidal Espana-Heredia˜ A Thesis Submitted to the Graduate Studies Office in Partial Fulfilment of the Requirements for the Degree of Master of Science in Aerospace Engineering Embry-Riddle Aeronautical University Daytona Beach, Florida Fall 2014 ERAU Luis E. Ferrer-Vidal Copyright by Luis Estefano Ferrer-Vidal Espana-Heredia˜ 2014 All Rights Reserved ii ERAU Luis E. Ferrer-Vidal Para mi abuelo y mi avi, Jorge y Santiago iv ERAU Luis E. Ferrer-Vidal Acknowledgments The author would like to thank his thesis advisor, Dr. Magdy Attia, for the help and ded- ication he provided through this investigation. The author is grateful for the amount of freedom and trust granted by Dr. Attia, allowing one to pursue this investigation in the manner one thought most appropriate, with adequate guidance when needed. Dr. Attia not only provided academic guidance, but also candid career advice which has proven invalu- able. The author would also like to thank Dr. Gangadharan and Dr. Ricklick for serving in this committee and reviewing the author’s work. They made themselves completely avail- able in helping the author in the final stages of this thesis. The help of Mr. Paul Johnson, NPSS modelling engineer at Wolverine Ventures, must also be acknowledged. Mr. Johnson’s help in developing the NPSS models proved essen- tial during the course of this work. The insights into pulse detonation modelling provided through discussions with Dr. Daniel Paxson, of NASA Glenn Research Center, are also ap- preciated, especially with regards to momentum averaging and lumped volume approaches. Special thanks are also due to the author’s parents and brother: Luis, Blanca, and Adolfo. Their support was essential during the span of the author’s academic career, serving as mo- tivation and a support structure without which no task would have been possible. To the author’s fiance, Priscila, much is owed for providing the much needed care and support throughout the past two years, playing an instrumental role in the author’s accomplish- ments. v ERAU Luis E. Ferrer-Vidal Abstract Author: Luis Estefano Ferrer-Vidal Espana-Heredia˜ Title: Investigation of Pulse Detonation Engine Flow Conditions for Turbomachinery Integration Institution: Embry-Riddle Aeronautical University Degree: Master of Science in Aerospace Engineering Year: 2014 Pressure gain combustion has been known to be more thermodynamically efficient than its constant pressure counterpart, which employs deflagration. Integration of pressure gain combustors into gas turbine engines has been and still is one of the most important chal- lenges facing the gas turbine industry. Pressure gain combustion devices are inherently unsteady. This unsteadiness affects turbomachinery components designed using steady op- eration assumptions, and results in mechanical inefficiencies which could outweigh the thermal efficiency benefits. Understanding of turbine components specifically made to operate in the exhaust conditions provided by a pressure gain combustor may yield new turbine design paradigms. As a first step towards the turbine design problem, this thesis discusses the effects of unsteady pressure gain combustion devices on gas turbine efficiency as well as current models for pulse detonation engine operation. A new simplified linear model for pulse detonation combustors is presented and compared to experimental data. This model is then implemented as an element in a cycle analysis code using Numerical Propulsion System Simulation (NPSS). This model captures the sharp peaks in flow prop- erty variation without the need for expensive computational resources, outperforming other simplified approaches in the literature. With this simplified model, tools may be developed to carry out performance studies of new turbine concepts for pressure gain combustors. vi Contents Acknowledgments v Abstract vi 1 Background 1 1.1 Problem Definition . .1 1.2 Current Technology . .3 1.3 Detonation Theory . .5 1.3.1 Chapman-Jouguet Detonations . .5 1.3.2 ZND Detonation Wave Structure . 10 1.4 Detonation for Turbo-Machines . 11 1.4.1 Thermodynamic Cycles . 11 1.4.2 Comparison of Cycle Efficiencies . 17 1.5 Pulse Detonation Flow Modelling . 18 2 Analysis 22 2.1 Flow Averaging Methods . 22 2.1.1 Exergy-Entropy Averaging . 23 2.1.2 Momentum Averaging . 26 2.2 Effects of Flow Unsteadiness on Turbine Performance . 29 2.2.1 Experimental Data Analysis . 29 vii ERAU Luis E. Ferrer-Vidal 2.2.2 Turbine Performance . 32 3 Modelling 37 3.1 Pulse Detonation Combustor Model . 37 3.1.1 Post-Detonation State . 37 3.1.2 Blowdown . 39 3.1.3 Exhaust . 43 3.1.4 Summary and limitations . 45 3.2 Plenum Modelling . 46 4 Simulation 50 4.1 NPSS Modelling . 50 4.1.1 Pulse Detonation Burner Element . 52 4.1.2 Pulse Detonation System Model . 53 4.1.3 Plenum Element . 54 4.1.4 Pulse Detonation Burner & Plenum System Model . 55 4.1.5 Pulse Detonation Burner & Turbine System Model . 56 4.2 NPSS Results . 57 4.2.1 Single Pulse Detonation Burner . 57 4.2.2 Pulse Detonation Burner & Plenum . 61 4.2.3 Pulse Detonation Burner & Axial Turbine . 64 5 Conclusions 66 5.1 Summary . 66 5.2 Future Work . 68 A One-Dimensional Similarity Flow Relations 74 B Control Volume Modelling for Plenum Element 79 viii ERAU Luis E. Ferrer-Vidal C NPSS Code Listings 81 C.1 Pulse Detonation Burner Element . 81 C.2 Plenum Element . 112 C.3 Model Files . 130 C.3.1 PlenumCheck.mdl . 130 C.3.2 PlenumCheck.run . 139 C.3.3 PDE Trb rig TRANS.run . 142 C.3.4 PDE lone.mdl . 146 C.4 Auxiliary Files . 163 ix List of Figures 1.1 Radial turbine - PDE rig used by the Air Force Research Laboratory [22]. .4 1.2 Control volume fixed on moving detonation wave. .6 1.3 Rankine-Hugoniot plot. .8 1.4 Structure of a ZND detonation wave [31]. 10 1.5 Idealized Brayton cycle. 12 1.6 Idealized Brayton and Humphrey cycles. 13 1.7 Step by step Fickett-Jacobs Cycle [31]. 14 1.8 Fickett-Jacobs Cycle Mollier Diagram. 15 1.9 Ideal PDE cycle Mollier Diagram. 17 1.10 Thermal efficiency of ideal PDE, Brayton and Humphrey cycles as function of initial compression static temperature ratio ( ) [8]. 18 1.11 Exit velocity vs. exited mass flow for simplified hybrid PDE model in red. Complete CFD results are presented in yellow while the blue and green represent two different algebraic models [16]. 20 1.12 Endo-Fujiwara Model Transient Pressure Variation [3]. 21 2.1 Exergy-Entropy equivalent states for total pressure (top) and total temper- ature (bottom). Data collected by Rouser [19]. 25 2.2 Momentum equivalent states for total pressure (top) and total temperature (bottom). Data collected by Rouser [19]. 28 x ERAU Luis E. Ferrer-Vidal 2.3 Pressure trace from ERAU Gas Turbine Laboratory Pulse Detonation Engine. 29 2.4 Pressure, temperature, and velocity data for 20 Hz ethylene PDE by Rouser [19]. 30 2.5 Cumulative enthalpy expelled from pulse detonation tube over two cycles. 31 2.6 Non-dimensional mass flow and enthalpy over two cycles. 32 2.7 Scaled turbine map for a generic low-sensibility turbine. 34 2.8 Scaled pressure ratio variation for PDE exhaust flow . 35 2.9 Efficiency variation for PDE exhaust flow . 35 3.1 Property variation vs similarity parameter. 40 3.2 Similarity rarefaction wave exiting a tube. 40 3.3 Similarity rarefaction wave following a detonation wave. 41 3.4 Arrangement of plenum and pulse detonation engine array. 47 4.1 Generic engine cycle model for NPSS analysis [14] . 51 4.2 NPSS model for a single pulse detonation engine. 54 4.3 NPSS model for PDE-plenum set-up. 55 4.4 NPSS model for PDE-turbine set-up. 56 4.5 Pressure trace for transient NPSS PDE model (Stoichiometric LPG-air). 58 4.6 Temperature trace for transient NPSS PDE model (Stoichiometric Ethene- air). 58 4.7 Mass flow trace for transient NPSS PDE model (Stoichiometric Ethene-air). 59 4.8 Comparison of experimental (red) and modelled (blue) pressure traces for single cycle PDE (Stoichiometric Ethene-air). 60 4.9 NPSS simulation of multiple pulses. 61 4.10 Massflow out of plenum over two cycles. 62 4.11 Temperature build-up in plenum over two cycles. 62 4.12 Pressure build-up in closed plenum due to PDE discharge. 63 xi ERAU Luis E. Ferrer-Vidal 4.13 Temperature build-up in closed plenum due to PDE discharge. 64 4.14 Variation of turbine efficiency and pressure ratio with time attached to a PDE in NPSS. 65 xii ERAU Luis E. Ferrer-Vidal Nomenclature γ adiabatic coefficient ηth thermal efficiency π pressure ratio ρ density τ characteristic time compression static temperature ratio A area B exergy Cp heat capacity at constant pressure cp specific heat capacity at constant pressure cv specific heat capacity at constant volume c sonic velocity d differential e specific internal energy H enthalpy h specific enthalpy L tube length M Mach number m mass m_ mass flow rate m_00 mass flux rate P pressure Q heat xiii ERAU Luis E.
Details
-
File Typepdf
-
Upload Time-
-
Content LanguagesEnglish
-
Upload UserAnonymous/Not logged-in
-
File Pages182 Page
-
File Size-