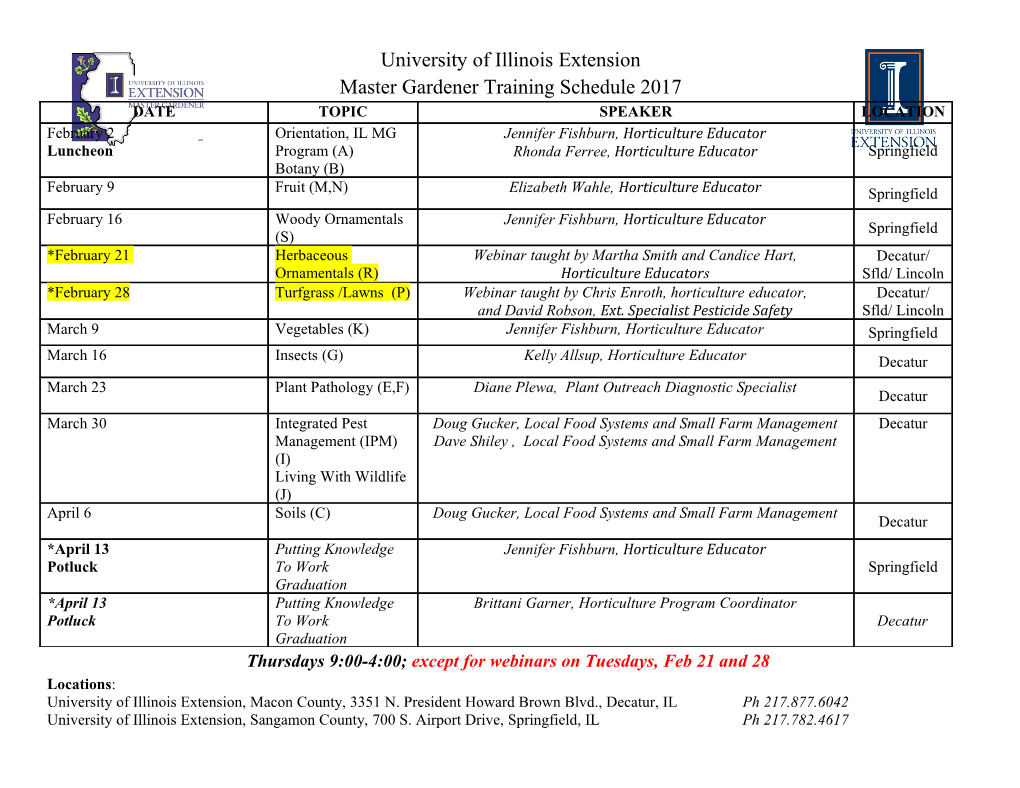
Preparation and cooling of magnesium ion crystals for sympathetic cooling of highly charged ions in a Penning trap Präparation und Kühlung von Magnesium-Ionenkristallen zum sympathetischen Kühlen von hoch geladenen Ionen in einer Penningfalle Vom Fachbereich Physik der Technischen Universität Darmstadt zur Erlangung des Grades eines Doktors der Naturwissenschaften (Dr. rer. nat.) genehmigte Dissertation von Tobias Murböck Dipl. Phys. aus Recklinghausen Tag der Einreichung: 23.11.2016, Tag der Prüfung: 12.12.2016 Darmstadt 2017 — D 17 1. Gutachten: Prof. Dr. Gerhard Birkl 2. Gutachten: Prof. Dr. Wilfried Nörtershäuser Preparation and cooling of magnesium ion crystals for sympathetic cooling of highly charged ions in a Penning trap Präparation und Kühlung von Magnesium-Ionenkristallen zum sympathetischen Kühlen von hoch geladenen Ionen in einer Penningfalle Genehmigte Dissertation von Tobias Murböck Dipl. Phys. aus Recklinghausen 1. Gutachten: Prof. Dr. Gerhard Birkl 2. Gutachten: Prof. Dr. Wilfried Nörtershäuser Tag der Einreichung: 23.11.2016 Tag der Prüfung: 12.12.2016 Darmstadt 2017 — D 17 Cover picture: Ion Coulomb crystal exhibiting several layers of singly charged magnesium ions. Bitte zitieren Sie dieses Dokument als: URN: urn:nbn:de:tuda-tuprints-60370 URL: http://tuprints.ulb.tu-darmstadt.de/6037 Dieses Dokument wird bereitgestellt von tuprints, E-Publishing-Service der TU Darmstadt http://tuprints.ulb.tu-darmstadt.de [email protected] Die Veröffentlichung steht unter folgender Creative Commons Lizenz: Namensnennung – Keine kommerzielle Nutzung – Keine Bearbeitung 4.0 International https://creativecommons.org/licenses/by-nc-nd/4.0 1 Contents 1 Motivation 3 1.1 Fine structure and hyperfine structure transitions . .6 1.1.1 Magnetic dipole transitions . .6 1.1.2 Fine structure transitions . .8 1.1.3 Hyperfine structure transitions . .9 2 The SpecTrap experimental setup 13 2.1 Production and transport of highly charged ions (HCI) . 15 2.1.1 The EBIS for production of HCI . 15 2.1.2 The HITRAP low-energy beamline . 17 2.1.3 The SpecTrap beamline . 20 2.2 The Mg+ ion source . 22 2.2.1 Ion creation . 22 2.2.2 Ion extraction . 23 2.2.3 Ion numbers and bunch structure . 25 2.3 The SpecTrap Penning trap system . 28 2.3.1 The magnet system . 28 2.3.2 Principles of charged particle trapping . 30 2.3.3 Single-ion motion in a Penning trap . 31 2.3.4 The Penning trap electrode design . 33 2.3.5 Expansion series for the potential of a cylindrical Penning trap . 35 i 2.3.6 Simulation of the coefficients Cn .................... 37 i 2.3.7 Measurements of the coefficients C2 .................. 39 i 2.3.8 Measurements of the coefficients C1 .................. 41 2.4 Electronic components . 42 2.4.1 Non-destructive ion detector (NID) . 42 2.4.2 Axial resonators for resistive cooling . 44 2.5 Experimental control and ion capture process . 50 2.5.1 The experiment control software . 50 2.5.2 The ion capture process . 52 2.5.3 Accumulation of ion bunches . 53 2.5.4 Ion capture with elevated potential or use of drift tube 2 . 54 2.6 Fluorescence detection . 56 2.6.1 The 280 nm laser system . 57 2.6.2 Fluorescence imaging and detectors . 57 2.6.3 Electronic transitions in 24Mg+ ..................... 59 i 2.6.4 Single-ion fluorescence rate . 61 3 Preparation of cold Mg+ clouds 65 3.1 Buffer gas cooling . 65 3.2 Laser Doppler cooling . 68 3.2.1 Laser Doppler cooling of a free ion . 69 3.2.2 Laser cooling of a trapped ion . 70 3.3 Combined buffer gas and laser cooling of Mg+ ................ 74 4 Single- and multi-species Coulomb crystals 81 4.1 Properties of non-neutral plasma in thermodynamic equilibrium . 83 4.2 The planar-shell model . 90 4.3 Observation of single-species ion crystals . 92 4.4 Multi-species ion crystals . 95 4.5 Sympathetic cooling and storage time of multiply charged ions . 101 5 Resonant-circuit measurements and a model for resistive cooling 105 5.1 Electronic ion detection with the resonant circuit . 105 5.2 Temporal evolution of resonant circuit signals . 108 5.3 A model for resistive cooling of large ion clouds . 110 5.3.1 Resistive cooling of a single ion . 111 5.3.2 Resistive cooling of an N-particle cloud . 111 5.3.3 Resistive cooling beyond the center-of-charge motion . 113 5.3.4 The rate equation for resistive cooling . 116 5.3.5 Thermalization by ion-ion collisions (Spitzer self-collision rate) . 117 5.3.6 Shifts of the axial frequency in a non-linear potential . 118 5.3.7 Comparison of the model with experimental signals . 121 6 Conclusion and outlook 129 A Additional material 133 A.1 QED contributions to atomic quantities . 133 A.2 Digital-trigger processing . 134 A.3 Parameters of the HITRAP and SpecTrap beamline . 135 A.4 Laser system, optical detection and atomic transitions . 140 A.5 Emission characteristic of an oscillating dipole . 142 A.6 The lineshape of atomic transitions . 142 A.7 Matrix element for electric dipole transitions . 144 B List of publications 145 Bibliography 147 ii Contents Abstract In this work, laser-cooled ion crystals containing 103 to 105 singly charged magne- sium ions (Mg+) were prepared in a Penning trap. The properties of the ion crystals and their structure displaying long-range ordering were analyzed by various non- destructive techniques. After creation of the Mg+ ions in the form of ion bunches in an external source, the ions were injected into the Penning trap where their temper- ature was reduced by eight orders of magnitude within seconds using a combination of buffer gas cooling and Doppler laser cooling. The achieved temperatures in the millikelvin-regime were close to the theoretical Doppler-cooling limit and sufficiently low to induce the transition to a crystal phase exhibiting long-range ordering. The structure of these mesoscopic ion crystals is in agreement with a model describing the crystal as a set of planar shells. This allows for a derivation of properties such as the charge density or the temperature of the observed crystals. For the process of combined buffer-gas and Doppler laser cooling an analytical model has been devel- oped, which explains the time development of the temperature and the fluorescence signal in agreement with the experimental results. The external ion source for the production of singly charged magnesium ions was developed and characterized. A SIMION simulation of the ion creation and extraction process allows to describe the ion bunch structure and to increase the Mg+ number by three orders of magnitude to 106 Mg+ ions per bunch. + + + + Other ion species with charge states between one (H2 ,C ,N2 , CO2 ) and three (Ar3+) were injected into the Mg+ crystals. Ion crystals containing more than one ion species were observed with structures in agreement with the theory of centrifugal separation, which indicates sympathetic cooling of the non-fluorescing ion species. This preparation of mixed ion crystals is an important step towards the sympathetic cooling of highly charged ions (HCI) and paves the way to future investigations of HCI, which will enable a stringent test of bound-state quantum electrodynamics. For supply of HCI to the SpecTrap experiment, the HITRAP low-energy beamline – including the pulsed drift tubes for ion deceleration and the electron beam ion source – were commissioned. Ion bunches containing several tens of thousands of argon ions (Ar13+) were transported to the SpecTrap Penning trap. These ion numbers are sufficient for future spectroscopy of the fine structure splitting in boron-like Ar13+. In future experiments, the HCI shall be delivered by HITRAP and will possess large kinetic energies immediate after capture into the Penning trap, so that initial sym- pathetic cooling is not very efficient. Therefore, the SpecTrap Penning trap features axial resonators for pre-cooling of the HCI to the kelvin-regime by means of resistive cooling. An analytical model for resistive cooling of highly charged ions was devel- oped in this work, which for the first time explained the measured resistive cooling signals of HCI given in literature. This model allows for a deeper understanding of the interaction between radial and axial degrees of freedom, it predicts the set of experimental parameters for optimum cooling, and it provides the time evolution of the HCI density and temperature during the cooling process. 1 Zusammenfassung Im Rahmen dieser Arbeit wurden in einer Penningfalle lasergekühlte Ionenkristalle mit Teilchenzahlen zwischen 103 und 105 Magnesiumionen (Mg+) erzeugt und die sich dabei ausbildenden Strukturen untersucht. Die Erzeugung der Ionenkristalle er- folgte dabei durch eine Kombination aus Puffergaskühlen und Laser-Dopplerkühlen, wodurch die Temperatur der Magnesiumionen innerhalb von wenigen Sekunden um acht Größenordnungen reduziert werden konnte. Die erreichte Temperatur der Mg+-Ionen war mit einigen Millikelvin hinreichend gering zur Ausbildung einer Kristall-Phase. Die Struktur der Ionenkristalle aus mehreren, zueinander paral- lelen Kristallschalen konnte in Übereinstimmung mit einem theoretischen Modell beschrieben werden. Aus der Modellierung der Ionenkristalle ließen sich weitere Kristalleigenchaften wie die Temperatur oder Ladungsdichte ableiten. Der Prozess des kombinierten Kühlens wurde im Rahmen eines theoretischen Modells analytisch beschrieben und wesentliche Eigenschaften des Kühlverlaufs konnten in Übereinstim- mung mit den experimentellen Befunden vorhergesagt werden. Zur Erzeugung der einfach geladenen Magnesiumionen wurde eine zweistufige Quelle, bestehend aus Magnesiumofen und Ionisationseinheit, entwickelt. In Über- einstimmung mit einer Simulation der Ionenerzeugung und Ionenextraktion konnte die Ionenquelle experimentell und theoretisch beschrieben werden sowie die Mg+- Produktion gegenüber einem vorherigen Design um mehr als drei Größenordnungen auf 106 Ionen pro Ionenpaket gesteigert werden. In die Kristalle aus lasergekühltem Magnesium wurden weitere, sowohl einfach als auch mehrfach geladene Ionen injiziert und die Ausbildung von Ionenkristallen bestehend aus mehreren Ionenspezies beobachtet.
Details
-
File Typepdf
-
Upload Time-
-
Content LanguagesEnglish
-
Upload UserAnonymous/Not logged-in
-
File Pages173 Page
-
File Size-