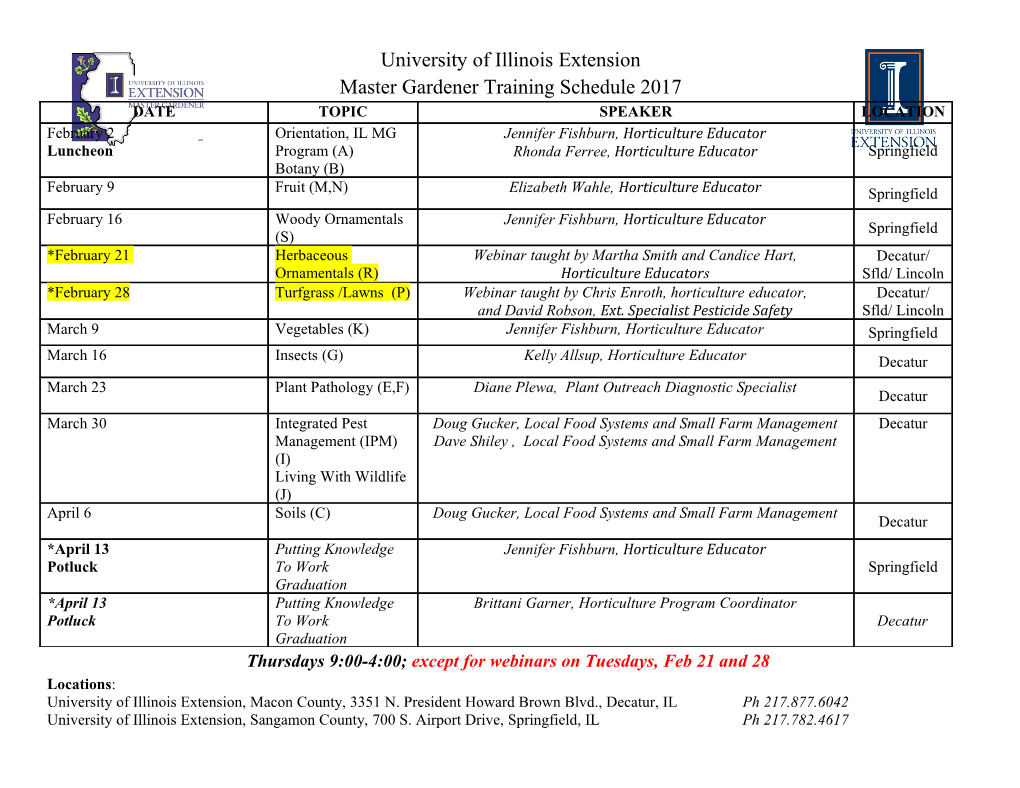
TheTheThe earlyearlyearly developmentdevelopmentdevelopment ofofof thethethe Earth,Earth,Earth, MoonMoonMoon andandand MarsMarsMars GIFTGIFT 20052005 TheHistoryofTheHistoryof theEarththeEarth EGU,EGU, ViennaVienna 26-2726-27thth AprilApril 2005 2005 Issues How are Earth-like planets built? How did silicate and metal reservoirs form? How did Earth acquire its volatile elements? Why isotope geochemistry ? Why isotope geochemistry ? Three main kinds of information: Rates and timing Tracing Past and present conditions Rates and timing Some significant radio-nuclides in the early solar system Nuclide Half-life (Myrs) Daughter 41Ca 0.1 41K 26Al 0.73 26Mg 60Fe 1.5 60Ni 53Mn 3.7 53Cr 107Pd 6.5 107Ag 182Hf 8.9 182W 247Cm 12 235U 205Pb 15 205Tl 129I16129Xe 92Nb 36 92Zr 244Pu 80 136Xe 146Sm 103 142Nd 235U704 207Pb 238U 4468 206Pb 87Rb 48800 87Sr Tracing (Hillson, 1986) Tracing 0.722 e. canine1 Sr 0.721 86 d. canine1 teeth Sr/ canine2, 87 0.720 1st, 2nd premolar (enamel) intestine 0.719 bone cortical 0.718 trabecular 0.717 0 1020304050 age [years] Past and present conditions Stable isotopes 18O 18O 16 sample − 16 std . δ 18O = O O x1000 18O std . 16O MultipleMultiple collectorcollector inductively inductively coupled coupled plasmaplasma source source massmass spectrometryspectrometry Opens the periodic table for isotopic research IA VIIIA Most of the world pre-1995 1 H II IIIA IVA VA VIA VIIA He 2 Li Be B C N O F Ne 3 Na Mg IIIB IVB VB VIB VIIB --- VIIIB --- IB IIB Al Si P S Cl Ar 4 K Ca Sc Ti V Cr Mn Fe Co Ni Cu Zn Ga Ge As Se Br Kr 5 Rb Sr Y Zr Nb Mo Tc Ru Rh Pd Ag Cd In Sn Sb Te I Xe 6 Cs Ba La Hf Ta W Re Os Ir Pt Au Hg Tl Pb Bi Po At Rn 7 Fr Ra Ac Ce Pr Nd Pm Sm Eu Gd Tb Dy Ho Er Tm Yb Lu Th Pa U Np Pu Am Cm Bk Cf Es Fm Md No Lr Unstable and Atmophile Lithophile Siderophile Chalcophile uncharacterised IA VIIIA Oxford developing… 1 H II IIIA IVA VA VIA VIIA He 2 Li Be B C N O F Ne 3 Na Mg IIIB IVB VB VIB VIIB --- VIIIB --- IB IIB Al Si P S Cl Ar 4 K Ca Sc Ti V Cr Mn Fe Co Ni Cu Zn Ga Ge As Se Br Kr 5 Rb Sr Y Zr Nb Mo Tc Ru Rh Pd Ag Cd In Sn Sb Te I Xe 6 Cs Ba La Hf Ta W Re Os Ir Pt Au Hg Tl Pb Bi Po At Rn 7 Fr Ra Ac Ce Pr Nd Pm Sm Eu Gd Tb Dy Ho Er Tm Yb Lu Th Pa U Np Pu Am Cm Bk Cf Es Fm Md No Lr Unstable and Atmophile Lithophile Siderophile Chalcophile uncharacterised Oxford developing… 1 H He 2 Li B C N O Ne 3 Mg Si S Cl Ar 4 K Ca Ti V Cr Fe Ni Cu Zn Ga Ge Se Br Kr 5 Rb Sr Zr Mo Ru Pd Ag Cd In Sn Sb Te Xe 6 Ba La Hf Ta W Re Os Ir Pt Hg Tl Pb Rn 7 Ra Ce Nd Sm Eu Gd Dy Er Yb Lu Th Pa U Atmophile Lithophile Siderophile Chalcophile ToTo taketake advantageadvantage ofof MCMC--ICPMSICPMS youyou needneed PhysicistsPhysicists whowho cancan designdesign andand buildbuild newnew kindskinds ofof MCMC--ICPMSICPMS instrumentsinstruments ToTo taketake advantageadvantage ofof MCMC--ICPMSICPMS youyou needneed ExpertsExperts inin laserlaser technologytechnology Issues How are Earth-like planets built? How did silicate and metal reservoirs form? How did Earth acquire its volatile elements? Planet formation Disk theory Star formation Astronomers can see stars like our Sun forming in the Orion Nebula Many of the new stars are embedded in a disk of dust and gas Dusty disks can also be detected around slightly older stars with interferometry. HR4796A is ~ 8 million years old Time-scales Saturn About 100 times bigger than the Earth Must have formed fast to trap nebular gases How did the Earth and Moon form? Ni others 4% 2% O30% Fe32% “Earth Pie” S3% Mg 14% Si15% Models for accreting the Earth Solar mass nebula (Cameron 1978) Minimum mass solar nebula (e.g. Hayashi 1978) Late stage collisions with no nebula (e.g. Wetherill 1986) The Moon probably formed from debris produced in a giant impact between the proto-Earth (~90% Earth mass) and another planet called "Theia" (~10% Earth mass). dust and rock debris... clumped together... ...and formed the Moon Model Time-scales for Accreting the Earth (>95%) Solar mass nebula < 106 yrs (Cameron 1978) Minimum mass solar nebula ~ 5 ×106 yrs (e.g. Hayashi 1978) Late stage collisions with no nebula 107 -108 yrs (e.g. Wetherill 1986) George Wetherill, 1986 Isotopes and time-scales 100 nucleosynthesis in stars % abundance remaining 0 0 collapse of solar nebula end of planetary growth start of planetary growth start of galaxy → time → present Isotopes and time-scales 100 nucleosynthesis in stars stable % abundance remaining 0 0 collapse of solar nebula end of planetary growth start of planetary growth start of galaxy → time → present Isotopes and time-scales 100 nucleosynthesis in stars stable long-lived % abundance remaining 0 0 collapse of solar nebula end of planetary growth start of planetary growth start of galaxy → time → present Some significant radio-nuclides in the early solar system Nuclide Half-life (Myrs) Daughter 41Ca 0.1 41K 26Al 0.73 26Mg 60Fe 1.5 60Ni 53Mn 3.7 53Cr 107Pd 6.5 107Ag 182Hf 8.9 182W 247Cm 12 235U 205Pb 15 205Tl 129I 16 129Xe 92Nb 36 92Zr 244Pu 80 136Xe 146Sm 103 142Nd 235U 704 207Pb 238U 4468 206Pb 87Rb 48800 87Sr 238238U/U/204204PbPb inin thethe earthearth 238 204 238 204 U/ Pbtotal earth = 5 × U/ Pbsolar system 238 204 U/ Pbtotal earth = 0.7 SilicateSilicate earthearth 238 204 corecore U/ Pbsilicate earth ~ 8 Pb-richPb-rich 238 204 U-poorU-poor U/ Pbcore = 0 U-rich,U-rich, Pb-poorPb-poor Clair Patterson 1956 Isotopes and time-scales 100 nucleosynthesis in stars stable long-lived % abundance remaining 0 0 collapse of solar nebula end of planetary growth start of planetary growth start of galaxy → time → present Isotopes and time-scales 100 nucleosynthesis in stars stable long-lived short-lived (extinct) % abundance remaining 0 0 collapse of solar nebula end of planetary growth start of planetary growth start of galaxy → time → present supernova remnant Cassiopeia A real stardust Some significant radio-nuclides in the early solar system Nuclide Half-life (Myrs) Daughter 41Ca 0.1 41K 26Al 0.73 26Mg 60Fe 1.5 60Ni 53Mn 3.7 53Cr 107Pd 6.5 107Ag 182Hf 8.9 182W 247Cm 12 235U 205Pb 15 205Tl 129I 16 129Xe 92Nb 36 92Zr 244Pu 80 136Xe 146Sm 103 142Nd 235U 704 207Pb 238U 4468 206Pb 87Rb 48800 87Sr Isotopes of hafnium and tungsten 40 Hafnium (Hf) 30 20 10 0 40 30 % Abundance 20 10 Tungsten (W) 0 174 176 178 180 182 184 186 Mass (amu) Isotopes of hafnium and tungsten 40 Hafnium (Hf) 30 182Hf ×1000 20 (extinct) 10 6 T½= 9 × 10 yrs 0 40 30 % Abundance 20 10 Tungsten (W) 0 174 176 178 180 182 184 186 Mass (amu) Isotopes of hafnium and tungsten 40 Hafnium (Hf) 30 182Hf ×1000 20 (extinct) 10 6 T½= 9 × 10 yrs 0 40 30 182W produced % Abundance 20 by decay ×1000 10 Tungsten (W) 0 174 176 178 180 182 184 186 Mass (amu) Hf/W in the Earth Hf/Wtotal Earth = Hf/Wsolar system = 1 SilicateSilicate EarthEarth corecore Hf/Wsilicate Earth = 15 W-richW-rich Hf-poorHf-poor Hf/Wcore = 0 Hf-rich,Hf-rich, W-poorW-poor NBNB SilicateSilicate EarthEarth == Earth'sEarth's PrimitivePrimitive MantleMantle Asteroid 4 Vesta - the source of eucrites? EucritesEucrites relative relative toto bulkbulk silicatesilicate EarthEarth Eucrites have a large Bouvante excess in 182W Millbillillie relative to BS Earth... Stannern Bereba BSE Juvinas Pasamonte Jonzac Serra de Mage Angra dos Reis -10 0 +10 +20 +30 +40 +50 ε182W (BSE) CarbonaceousCarbonaceous chondrites...chondrites... ...represent...represent bulkbulk solarsolar systemsystem EucritesEucrites relative relative toto bulkbulk solarsolar systemsystem Bulk solar system is Bouvante 182 Millbillillie slightly lower in W ……thereforetherefore than BS Earth (Kleine Stannern and others 2002) AsteroidAsteroid 44 VestaVesta Bereba accretedaccreted andand BSE differentiateddifferentiated Juvinas BSS Pasamonte overover aa shortershorter Jonzac timetime frameframe thanthan EarthEarth Serra de Mage Angra dos Reis -10 0 +10 +20 +30 +40 +50 ε182W (BSE) Some eucrites thought to come from Asteroid 4 Vesta Earliest formed objects -calcium aluminum refractory inclusions 0 20 40 60 Time after start of solar system (millions of years) EucritesEucrites relative relative toto bulkbulk solarsolar systemsystem Bulk solar system is Bouvante slightly lower in 182W Millbillillie than BS Earth (Kleine Stannern and others 2002) …what…what doesdoes Bereba thisthis meanmean forfor BSE Juvinas the time-scale BSS the time-scale Pasamonte ofof EarthEarth Jonzac accretion?accretion? Serra de Mage Angra dos Reis -10 0 +10 +20 +30 +40 +50 ε182W (BSE) George Wetherill, 1986 Accretion of the Earth 1.0 τ = 10 0.8 13 17 25 0.6 50 100 0.4 0.2 Wetherill 1986 Mass fraction of earth τ = accretionary mean life (Myrs) 0 0 20 40 60 80 100 Time (Myrs) Continuous core formation ÎÎ Gradual accretion, mixing, isotopic equilibration and metal segregation The ~ 2 εW excess of the silicate Earth relative to average solar system is consistent with ~63% accretion in 11 Myrs τ Earth Some eucrites thought to come from Asteroid 4 Vesta Earliest formed objects -calcium aluminum refractory inclusions 0 20 40 60 Time after start of solar system (millions of years) Model Time-scales for Accreting the Earth (>95%) Solar mass nebula < 106 yrs (Cameron 1978) Minimum mass solar nebula ~ 5 ×106 yrs (e.g.
Details
-
File Typepdf
-
Upload Time-
-
Content LanguagesEnglish
-
Upload UserAnonymous/Not logged-in
-
File Pages113 Page
-
File Size-