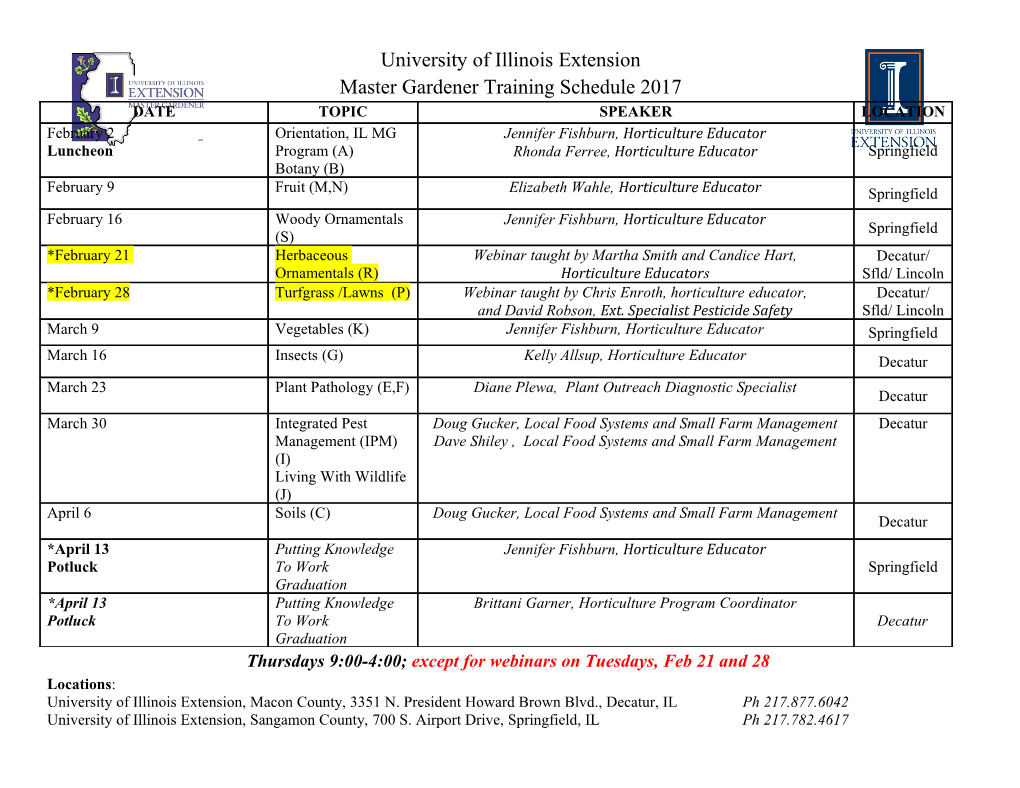
BI80CH19-McHenry ARI 16 May 2011 14:39 DNA Replicases from a Bacterial Perspective Charles S. McHenry Department of Chemistry and Biochemistry, University of Colorado, Boulder, Colorado 80309; email: [email protected] Annu. Rev. Biochem. 2011. 80:403–36 Keywords The Annual Review of Biochemistry is online at biochem.annualreviews.org DNA polymerase III, DnaX complex, processivity, error-prone polymerase, PHP exonuclease This article’s doi: 10.1146/annurev-biochem-061208-091655 Abstract Copyright c 2011 by Annual Reviews. All rights reserved Bacterial replicases are complex, tripartite replicative machines. They by University of Colorado - Boulder on 06/17/11. For personal use only. contain a polymerase, polymerase III (Pol III), a β2 processivity factor, 0066-4154/11/0707-0403$20.00 β Annu. Rev. Biochem. 2011.80:403-436. Downloaded from www.annualreviews.org and a DnaX complex ATPase that loads 2 onto DNA and chaperones Pol III onto the newly loaded β2. Bacterial replicases are highly proces- sive, yet cycle rapidly during Okazaki fragment synthesis in a regulated way. Many bacteria encode both a full-length τ and a shorter γ form of DnaX by a variety of mechanisms. γ appears to be uniquely placed in a single position relative to two τ protomers in a pentameric ring. The polymerase catalytic subunit of Pol III, α, contains a PHP domain that not only binds to a prototypical ε Mg2+-dependent exonuclease, but also contains a second Zn2+-dependent proofreading exonuclease, at least in some bacteria. This review focuses on a critical evaluation of re- cent literature and concepts pertaining to the above issues and suggests specific areas that require further investigation. 403 BI80CH19-McHenry ARI 16 May 2011 14:39 Contents 4.5. The Rate of Polymerase 1.INTRODUCTION.............. 404 Dissociation upon Collision 2. INITIATION COMPLEX with the Preceding Primer Is FORMATION................... 406 Too Slow to Support Okazaki Fragment Synthesis. 419 2.1. The β2 Clamp-Loading Reaction and the DnaX 4.6. ATP Hydrolysis by an τ Complex...................... 406 Associated -Containing DnaX Complex Might Be Part of the 2.2. Mechanism of β2 Loading on PrimedDNA................. 406 Circuit for Polymerase 2.3. Chaperoning of Polymerase III Cycling....................... 420 4.7. Primase-Polymerase III onto Newly Loaded β2 by the DnaXComplex............... 408 Handoff....................... 420 2.4. Hydrolysis of an ATP by Each 4.8. Is the Asymmetry of the DnaX Protomer May Not Be Polymerase III Holoenzyme Required for Initiation Relevant to the Recycling Complex Formation . 410 Issue? . 420 2.5. Does the DnaX Complex 5. DNAX COMPLEX COMPOSITION AND Really Open the β2 Clamp? . 410 3.ELONGATION................. 411 ASSEMBLY WITHIN CELLS. 421 τ γ 3.1. Interactions that Contribute to 5.1. and Composition of Polymerase III Processivity . 411 Authentic DnaX Complex . 421 3.2. DNA Polymerase 5.2. DnaX Complex Assembly. 423 IIIStructure.................. 412 6. PROOFREADING WITHIN A 3.3. Structure of Proteins REPLICASE THAT MAY Associated with CONTAIN TWO DISTINCT PolymeraseIII................ 416 EDITING EXONUCLEASES . 424 4. CYCLING OF THE 6.1. Structure, Function, and ε LAGGING-STRAND Interactions of , the Major REPLICASE DURING Proofreading Subunit . 424 OKAZAKI FRAGMENT 6.2. The PHP Domain of α SYNTHESIS.................... 416 Polymerase III , a Second 4.1. Competing Models for Proofreading Activity? . 425 by University of Colorado - Boulder on 06/17/11. For personal use only. ReplicaseCycling............. 416 7. EMERGING AREAS OF 4.2. Cycling Models Provided by INTEREST: BACTERIA WITH Annu. Rev. Biochem. 2011.80:403-436. Downloaded from www.annualreviews.org Bacteriophages T4 and T7 . 417 MULTIPLE POLYMERASE IIIS 4.3. Does the OB Fold Provide the AND THE CHEMICAL Processivity Switch BIOLOGY OF DNA forCycling?................... 418 REPLICATION................. 426 4.4. What Is τ’s Role inCycling?.................... 418 1. INTRODUCTION bacteria and Pol δ and ε in eukaryotes, a slid- Cellular chromosomal replicases from all ing clamp processivity factor (β2 in bacteria branches of life are tripartite. They contain a and proliferating cell nuclear antigen in eukary- polymerase, DNA polymerase III (Pol III) in otes), and a clamp loader (DnaX complex in 404 McHenry BI80CH19-McHenry ARI 16 May 2011 14:39 bacteria and replication factor C in eukaryotes). along the lagging-strand template (4). The ex- By themselves, replicative polymerases do not posed single-stranded DNA (ssDNA) is coated exhibit special properties that distinguish them on the lagging strand by a ssDNA-binding pro- SSB: single-stranded from other polymerases, but together with the tein (SSB in bacteria or a heterotrimeric repli- DNA-binding protein sliding clamp and clamp loader, they become cation protein A in eukaryotes). Priming is cat- Pol III HE: highly processive (1). Early functional stud- alyzed by a dedicated primase in all systems, DNA polymerase III ies revealed β2 as the key processivity factor generating short RNA primers that are elon- holoenzyme (2), and an ensuing crystal structure elegantly gated by a DNA polymerase (Figure 1a). showed the structural basis for its function (3). In this review, issues pertaining to bacterial β2 forms a ring that surrounds the DNA tem- replicases are examined using the E. coli DNA plate and tethers the polymerase to it, much polymerase III holoenzyme (Pol III HE) as like a carabiner surrounds a rope and tethers a a prototype, except where differences are rock climber so that any transient dissociation understood and viewed as important. Topics is reversible, enabling processive replication. were chosen for inclusion either because they Other features of bacterial replication are relatively new and not covered by other (Figure 1) are similarly conserved among all reviews or because new ways of looking at life forms. A hexameric helicase precedes other some old problems are considered. I have taken replication functions and separates two strands the approach of reevaluating concepts that lack at the replication fork. In bacteria, the helicase full support and, where alternatives exist, that → (DnaB6 in Escherichia coli ) translocates 5 3 have not been fully considered. My intent is acb 5' ' DnaX complex β 3 2 RNA E DnaG SSB Pol III primer D δ' A primase χ ψ γ δ Primase DnaXCX ττ SSB C B DnaB χ 3' β β helicase ψ αεθ 5' 2 2 δ' δ τ β Helicase γ α α αεθ τ ε ε Pol III β 5' θ θ by University of Colorado - Boulder on 06/17/11. For personal use only. 3' Annu. Rev. Biochem. 2011.80:403-436. Downloaded from www.annualreviews.org Figure 1 DNA polymerase III holoenzyme (Pol III HE) contacts at the replication fork. (a) A hexameric helicase (DnaB in E. coli )usesthe energy of ATP hydrolysis to translocate down the lagging-strand template, splitting two strands apart in advance of the leading-strand replicase, Pol III HE. Single-stranded regions of the lagging-strand template are coated by ssDNA-binding protein (SSB). Primase interacts with the helicase and synthesizes short RNA primers for Okazaki fragment synthesis; these primers are extended by the Pol III HE until a signal is received to recycle to the next primer synthesized at the replication fork (Section 4). Gaps between Okazaki fragments are filled, RNA primers are excised by DNA polymerase I, and the resulting nicks are sealed by a DNA ligase (not shown). For clarity, this view is drawn with discrete DnaXcx on each Pol III; they are actually shared between the leading- and lagging-strand polymerase (dashed line). (b) Details of known subunit interactions within the Pol III HE. ψ threads through all three DnaX protomers (45) and is shown here to signify the unique cross-link observed with γ (46) (Section 2.2). In addition, there is a transient interaction between δ and, perhaps, additional DnaXcx subunits with β2 during the clamp-loading reaction. The subunit positions (A, B, C, D, and E) are indicated using the scheme suggested by M. O’Donnell. (c) A cartoon of the replication fork showing relevant protein-protein interactions, including dimerization of the leading- and lagging-strand polymerases through contact of domain V of τ with α (24). A contact between domain IV of two τs and two DnaB protomers anchors the replicase to the helicase, placing all replication fork components into one replisome (25, 70). www.annualreviews.org • Bacterial Replicases 405 BI80CH19-McHenry ARI 16 May 2011 14:39 to stimulate experimentation that will provide The clamp loader was initially named after a firm foundation for correct concepts or lead the DnaX γ-subunit (32) before τ was discov- to consideration of new paradigms. There are ered (33) and later shown to also be a product of excellent reviews that cover historical and other the dnaX gene (34). The literature often refers issues more completely than I do here (5–10). to the clamp loader as the γ complex (γcx), a term that can be confusing because clamp load- ers can be constituted that do not contain γ, 2. INITIATION COMPLEX only τ. We use the general term DnaX com- FORMATION plex (DnaXcx) to refer to the clamp loader and use the term τ complex (τ ) when we are specif- 2.1. The β Clamp-Loading Reaction cx 2 ically referring to DnaX that lacks γ. and the DnaX Complex cx Before DNA elongation begins, the Pol III HE forms an initiation complex in an ATP- 2.2. Mechanism of β2 Loading on dependent reaction (11–14). This reaction is of- Primed DNA ten artificially divided into an ATP-dependent The basic models of the first step of initia- clamp-loading reaction in which a β2 ring is tion complex formation, clamp loading, have loaded around DNA and a subsequent associ- been worked out by the O’Donnell labora- ation of DNA Pol III occurs with the loaded tory in a long-standing productive collabora- clamp (13–15).
Details
-
File Typepdf
-
Upload Time-
-
Content LanguagesEnglish
-
Upload UserAnonymous/Not logged-in
-
File Pages38 Page
-
File Size-