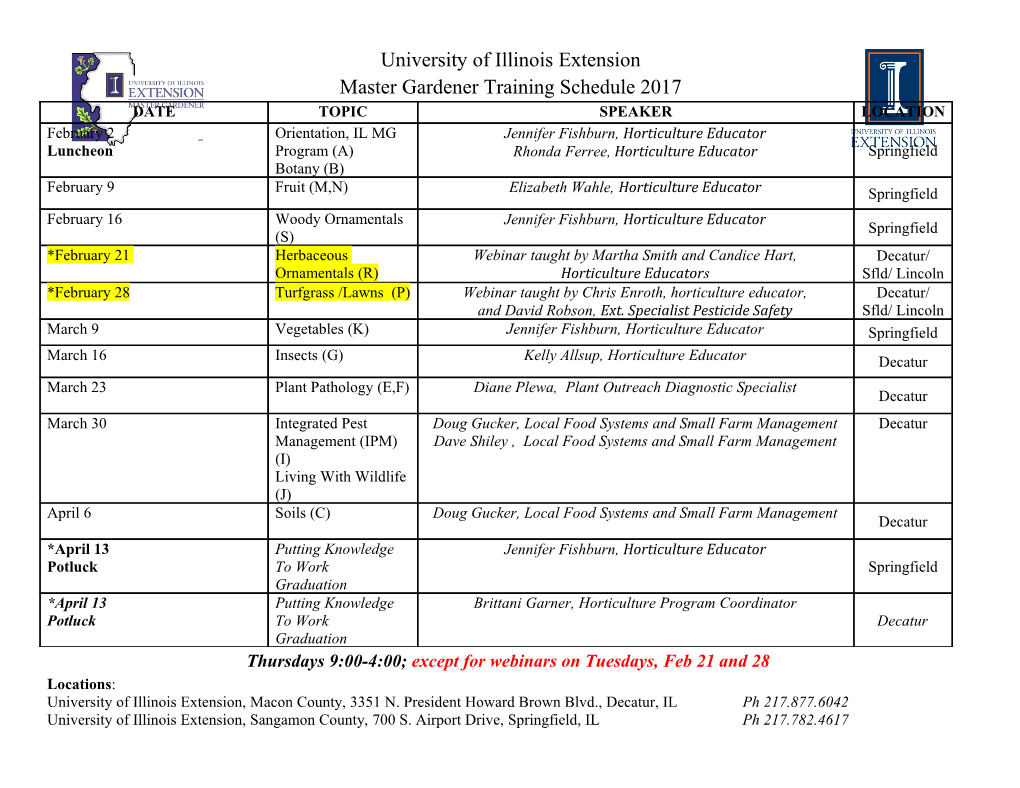
Structure and Dynamics by Experiment and Theory: Concerted Applications of Gas Electron Diffraction and Computational Chemistry Conor Douglas Rankine Doctor of Philosophy University of York Chemistry June 2019 To everyone in the C/A/057 Phys. Chem. Office: “Well …whatever [your plan] was, I’m sure it was better than my plan to get out of all this by pretending to be mad. I mean, who would have noticed another madman ‘round here?” Edmund Blackadder. “Goodbyeee”. Blackadder Goes Forth. BBC. 2 Nov. 1989. ii Abstract To plan and prepare the strongest research proposals for time-resolved gas electron diffraction (TRGED) experiments, the author has launched and overseen the development of two new research programmes in the Wann Electron Diffraction Group. A time-averaged gas electron diffraction (GED) programme has seen the technique re- established in the UK following the relocation, recommission, and modernisation of a 1960s gas electron diffractometer. Two case studies – a) 4-(dimethylamino)benzonitrile, and b) tinII bis(trifluoroacetate), ditinII μ-oxy-bis-μ-trifluoroacetate, and tinIV tetrakis(trifluoroacetate) – highlight the range of chemical samples that are accessible to study using the upgraded gas electron diffractometer. A computational chemistry programme has seen trajectory surface-hopping dynamics (TSHD) introduced to the Wann Electron Diffraction Group, delivering a paradigm shift in the ability of the research group to plan and interpret TRGED experiments. Parallel Python code has been developed to simulate TRGED data and benchmarked with up to 64 CPU cores as part of this programme. High performance is achieved in the strong and weak parallel scaling regimes. The interplay between the two programmes is illustrated in three case studies: the photolysis of 1,2-diiodotetrafluoroethane, the photofission of the disulfide bond in 1,2-dithiane, and the photoisomerisation of E-cinnamonitrile. The photolysis of 1,2-diiodotetrafluoroethane is found to take place on the triplet excited- state manifold, and statistical analysis has revealed that secondary dissociation of I• from the primary photolysis product is more likely following primary photolysis of the antiperiplanar (as opposed to the synperiplanar) isomer of 1,2-diiodotetrafluoroethane. A transient bridged intermediate has been characterised for the first time at the intersection of the D1 and D0 states; the intermediate may appear in less than 100 fs post-photolysis. The photofission of the disulfide bond in 1,2-dithiane is found to trigger a classically- intuitive “Molecular Clackers” mechanism that couples the S1 and S0 states, challenging contemporary understanding of the origin of the photostability of 1,2-dithiane. The “Molecular Clackers” mechanism drives periodic collisions between the termini of a transient thiyl biradical that can result in S0 ← S1 internal conversion and the permanent recoupling of the termini, repairing the broken disulfide bond on the picosecond timescale. The photoisomerisation of E-cinnamonitrile is revealed to be wavelength-dependent, and several key S0 ← S1 internal conversion pathways have been characterised for the first time. iii Contents Abstract ................................................................................................................................. iii Contents ..................................................................................................................................iv List of Figures ........................................................................................................................ix List of Tables ........................................................................................................................ xxi Acknowledgements ............................................................................................................ xxiv Authorship Declaration ....................................................................................................... xxv 1 Motivation: Time-Resolved Gas Electron Diffraction .................................................... 1 1.1 Photochemistry, and “Photographing in the Dark” ................................................. 1 1.2 Time-Resolved Electron Diffraction ........................................................................ 4 1.2.1 What Is Time-Resolved Gas Electron Diffraction?.......................................... 4 1.2.2 Why This? ........................................................................................................ 5 1.2.3 Why Now? ........................................................................................................ 7 1.3 Electron Diffraction 1924 – 2019 ............................................................................ 8 1.4 Next-Generation Time-Resolved Electron Diffraction Experiments ..................... 16 1.5 Structure of the Thesis ........................................................................................... 19 2 Theory I: Time-Averaged Gas Electron Diffraction ..................................................... 21 2.1 Gas Electron Diffraction ........................................................................................ 21 2.2 Molecular Intensity and Radial Distribution Curves ............................................. 25 2.3 Refinement ............................................................................................................. 27 2.4 Gas Electron Diffractometers................................................................................. 29 2.4.1 Electron Source .............................................................................................. 29 2.4.2 Sample Input................................................................................................... 30 2.4.3 Electron Detection .......................................................................................... 30 2.5 Challenges and Limitations in Gas Electron Diffraction ....................................... 32 2.5.1 Volatilisation .................................................................................................. 32 iv 2.5.2 Correcting for Molecular Vibrations ............................................................. 32 3 Theory II: Ab Initio Computational Chemistry and Trajectory Surface-Hopping Dynamics .............................................................................................................................. 35 3.1 Schrödinger Equation ............................................................................................ 35 3.2 Born-Oppenheimer Approximation ...................................................................... 37 3.3 Breakdown of the Born-Oppenheimer Approximation ......................................... 38 3.4 Single- and Multireference Wavefunctions ........................................................... 40 3.5 Trajectory Surface-Hopping Dynamics ................................................................. 42 3.5.1 Initial Conditions ........................................................................................... 43 3.5.2 Propagation .................................................................................................... 43 3.5.3 Decoherence Correction ................................................................................ 44 3.5.4 Surface-Hopping ............................................................................................ 45 3.5.5 Practical Considerations in Trajectory Surface-Hopping Dynamics ............. 46 4 New Methods & Applications I: A New Lease of Life for the Only Gas Electron Diffractometer in the UK ...................................................................................................... 49 4.1 University of York Gas Electron Diffractometer .................................................. 50 4.1.1 Vacuum System ............................................................................................. 51 4.1.2 Electron Source .............................................................................................. 51 4.1.3 Electron Optics .............................................................................................. 52 4.1.4 Sample Input .................................................................................................. 52 4.1.5 Electron Detection ......................................................................................... 54 4.2 Gas-Phase Structure of 4-(Dimethylamino)benzonitrile ....................................... 56 4.2.1 4-(Dimethyl)aminobenzonitrile ..................................................................... 56 4.2.2 Data Acquisition ............................................................................................ 57 4.2.3 Density Functional Theory Calculations ....................................................... 57 4.2.4 Data Reduction and Refinement .................................................................... 58 4.2.5 Discussion ...................................................................................................... 61 4.3 Gas-Phase Structures of Fluorine-Doped Tin Oxide Precursors .......................... 65 v 4.3.1 Fluorine-Doped Tin Oxide Precursors ........................................................... 65 4.3.2 Data Acquisition ............................................................................................. 66 4.3.3 Density Functional Theory Calculations .......................................................
Details
-
File Typepdf
-
Upload Time-
-
Content LanguagesEnglish
-
Upload UserAnonymous/Not logged-in
-
File Pages323 Page
-
File Size-