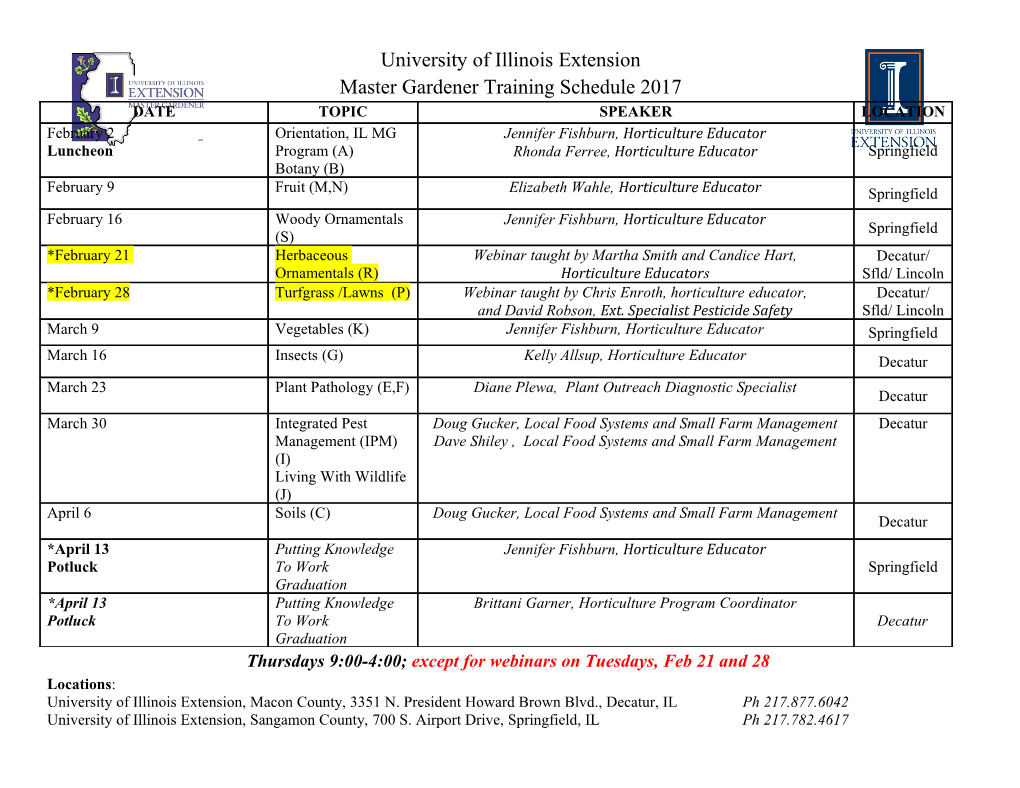
Hyperfine Interact DOI 10.1007/s10751-014-1121-9 Muons as hyperfine interaction probes in chemistry Khashayar Ghandi · Amy MacLean © Springer International Publishing Switzerland 2015 Abstract Spin polarized positive muons injected in matter serve as magnetic probes for the investigation of physical and chemical properties of free radicals, mechanisms of free radical reactions and their formations, and radiation effects. All muon techniques rely on the evolution of spin polarization (of the muon) and in that respect are similar to conventional magnetic resonance techniques. The applications of the muon as a hyperfine probe in several fields in chemistry are described. Keywords Free radicals · Muonium · Muon · Radiation chemistry · Hyperfine interactions Outline In designing new materials and chemical processes, it is of paramount importance to understand what is happening at an atomic level. Muon techniques provide a unique glance at such microscopic properties, given the kinetic time window which they can probe, as well as their extreme sensitivity to local magnetic and electronic environments. The applications of muon spectroscopy are far reaching, from nuclear reactor development to targeted can- cer treatments to green industrial processes. Many and more of these applications will be discussed in this brief review. This review paper includes the following: 1) A very brief review of the muon with references to muon literature. 2) An explanation of the muon as a probe of free radical kinetics. 3) The futuristic aspects of the muon as a probe of free radical kinetics. 4) The muon as a hyperfine probe of hydrogen atoms in different materials. 5) The muon as a probe of the mechanisms of free radical reactions. Proceedings of the 5th Joint International Conference on Hyperfine Interactions and International Symposium on Nuclear Quadrupole Interactions (HFI/NQI 2014), Canberra, Australia, 21–26 September 2014 K. Ghandi () · A. MacLean Department of Chemistry & Biochemistry, Mount Allison University, Sackville, NB, Canada e-mail: [email protected] K. Ghandi, A. MacLean Fig. 1 Muonium (Mu) from a chemical perspective is considered an isotope of the H atom. Electronic properties of Mu and the H atom are compared here This review is by no means all-inclusive and it only summarizes some of the represen- tative recent works in the above list. For a more detailed review of the muon and muon techniques, see ref [1]. Several recent reviews referred to in [1] provide comprehensive overviews of other aspects not covered in this review (such as applications to soft matter and surfaces). 1 The positive muon, muonium, and muoniated free radicals The positive muon, μ+, is an elementary particle that, in the context of this review, is best regarded as a light proton with one-ninth the proton mass. Like the proton, it is a spin-1/2 particle. Its magnetic moment is 3.18334 larger than the magnetic moment of the proton, making it useful as a magnetic probe of matter. Muon spectroscopy techniques are based on muon spin evolution in the presence of transverse or longitudinal magnetic fields, rel- ative to the direction of the muon beam spin. In this edition of Hyperfine Interactions, Brewer gives a historical review of the muon, its applications as a probe of magnetic and electronic environments in material, and different muon techniques [1]. We would refer the reader to this review article for a more in-depth examination of the muon techniques. In the chemical probe applications discussed here, information is extracted from the ini- tial amplitude and the spin evolution of the muon in different magnetic and electronic environments [1]. After a muon is injected into a material, one of several events can occur. The muon can remain in its diamagnetic state, either as a molecular ion or diamagnetic neutral species [2], but it can also capture an electron from its radiation track to form paramagnetic muo- nium (Mu). Mu can be considered an ultra-light isotope of the H atom (Fig. 1), because Muons as hyperfine interaction probes in chemistry within the Born-Oppenheimer approximation, the electronic properties (and hence chemical properties) of a single electron atom depend on its reduced mass (1). mμme mr = ≈ me (1) mμ + me Mu has one-ninth the mass of H and, therefore, significant isotope effects can be expected for different reactions. This will be discussed later in the context of applications for investigating chemical dynamics. The short lifetime of muon, 2.2 μs, makes it useful for studying fast kinetic processes involving the H-atom with pseudo first order rate constants on the order of 105 to 107 s−1 [2]. The in situ formation of muonium in the presence of other reactants often leads to the formation of more complicated and interesting free radicals. There are four ways that a muoniated free radical can be formed: 1) Addition of Mu to a molecule with a double bond (2): C6H6 + Mu → C6H6Mu (2) 2) Reaction of a solvated electron and a muoniated molecular ion (3): + − C6H6Mu + e(sol) → C6H6Mu (3) 3) Reaction of Mu and a molecule with carbene or carbene-like functional groups [3]. 4) Dissociation of a reactive intermediate in its vibrational excited state [4]. In addition to the pathways listed above, muoniated free radicals can go on to react with other reagents in the system; this will be further discussed later. Positrons from the decay of muons in situ must first be detected to learn about reaction kinetics, free radical formation and related free radical mechanisms using muon techniques, positrons from decay of muons must first be detected. Indeed, the positrons can easily pass through solid walls and be detected external to the reaction vessel. The relevant spin, magnetic precession and hyperfine coupling information carried in the decay products can then be measured to study materials. The combination of techniques used to study muo- niated free radicals or muon methods in molecular and material science are collectively called μSR [1]. There are three main muon techniques utilized in chemistry. The first, TF-μSR (trans- verse field μSR), involves the application of a magnetic field perpendicular to the direction of spin, and is most useful for studying reaction rates and muon-electron hyperfine interac- tions. The second, LF-μSR (longitudinal field μSR), is often used to study spin dynamics, though it can also identify free radicals formed either in slow reactions or at low precursor concentration. In both cases, a magnetic field parallel to the muon beam spin is applied. In the case of either low concentration of the precursor or slowly formed free radicals, the TF-μSR spectra is lost due to dephasing of spin polarization [1]. One type of LF method is Radio Frequency-μSR (RF-μSR), in which radio frequency is applied perpendicular to muon spin, in addition to the LF. This is a resonance method similar to NMR. Another type of LF muon method is Avoided Level Crossing Resonance (ALCR) [1], which is also a res- onance technique. In isotropic environments, ALCR provides isotropic hyperfine coupling constants for spin nuclei other than muon, and in anisotropic environments, it provides infor- mation on free radicals bound anisotropically via hyperfine coupling of muon and electron [1–4]. Finally, in ZF-μSR (zero field -μSR), the only magnetic influences on the muon arise from the sample itself; even the earth’s magnetic field is cancelled out. This can be used to study the intrinsic magnetic properties of materials [1]. K. Ghandi, A. MacLean Fig. 2 Splitting of spin states of muonium (Mu) (H) in a magnetic field. The spin states on the left side show the low field states where mixing of states happens. The spin states on the right side show the high field states. Most Mu studies in the TF use low field conditions. The two transitions typically used to measure the hyperfine coupling constant (HFC) of Mu in TF are also shown 2 Muon as a probe of free radical kinetics Due to the volume of research that has been done on free radical kinetics using muon meth- ods, this section is divided into two parts. First, we will review muon-based kinetic studies in the gas phase, followed by a section on condensed phase studies using the same methods. 2.1 Gas phase studies Hydrogen is the simplest atom in nature. Consequently, the study of its interactions and chemical reaction rate constants (kH ) has been central to the field of reaction dynamics since the studies of the first potential energy surface (PES) for H + H2 [5, 6]. In the realm of reactive collision dynamics, modern atomic and molecular beam studies of H atom reac- tions produced under near single-collision conditions at epithermal (∼ 1 eV) energies, with their ability to probe ro-vibrational cross sections at the state-to-state level, play an ever increasingly important role in assessing reaction rate theories [7, 8]. However, as these col- lision impact energies are well above threshold, the general features of reactive collision processes are often well described by classical (or quasi-classical) dynamics. Therefore, the study of chemical kinetics and thermal rate constants, which are generally much more sen- sitive to quantum mass effects in probing the details of the PES at the barrier, continue to be important. This is all the more true in the case of a sensitive isotopic mass probe, such as Mu [9–15]. Kinetic studies of chemical reactions most often require measurements of the Mu decay rate using the (TF-μSR) technique at low magnetic fields (2−10 G) [1]. This can be done both at continuous beam facilities, such as TRIUMF (Canada) or PSI (Switzerland) for faster reactions (equivalent to the first order rate constants in the range of 1 to 4 μs−1),or at pulsed facilities, such as the Rutherford Appleton Laboratory (UK) and JPARC (Japan), for slower reactions (with first order rate constants smaller than 1 μs−1).
Details
-
File Typepdf
-
Upload Time-
-
Content LanguagesEnglish
-
Upload UserAnonymous/Not logged-in
-
File Pages18 Page
-
File Size-