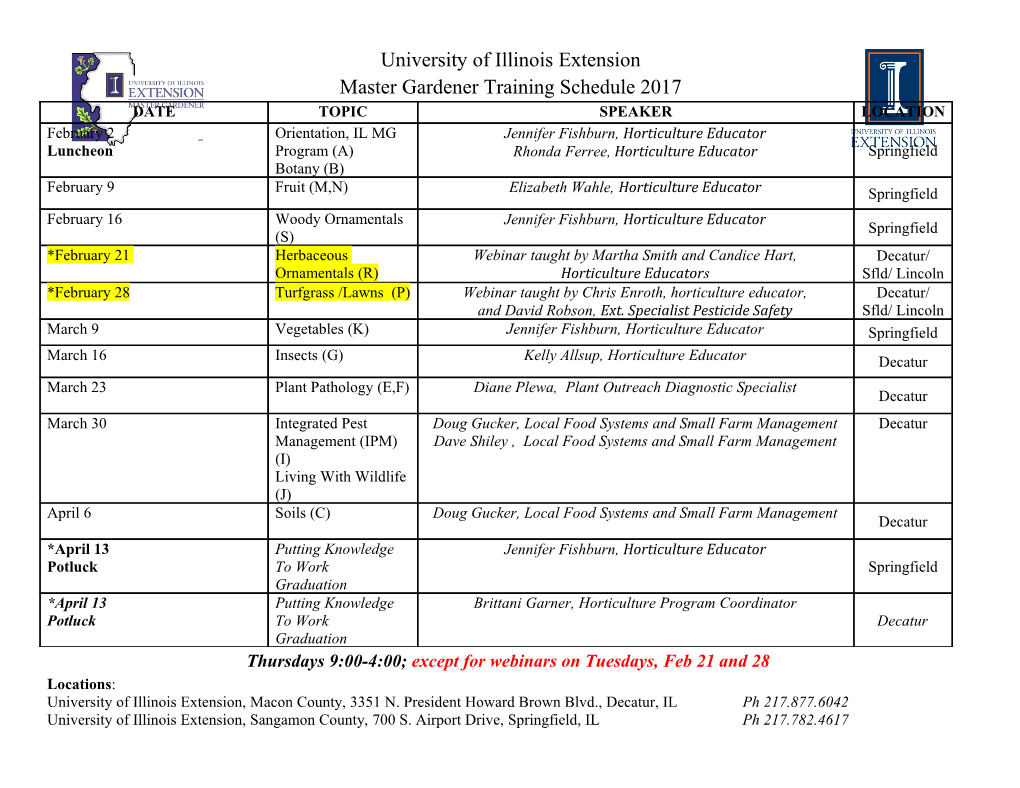
NUCLEAR TANKER PRODUCING LIQUID FUELS FROM AIR AND WATER By JOHN MICHAEL GALLE-BISHOP B.S. English (Honors) United States Naval Academy 2002 M.A. English George Mason University 2003 M.E.M. Old Dominion University 2008 Submitted to the DEPARTMENT OF NUCLEAR SCIENCE AND ENGINEERING And the DEPARTMENT OF MECHANICAL ENGINEERING In Partial Fulfillment of the Degrees of MASTER OF SCIENCE IN NUCLEAR SCIENCE AND ENGINEERING And MASTER OF SCIENCE IN NAVAL ARCHITECTURE AND MARINE ENGINEERING At the MASSACHUSETTS INSTITUTE OF TECHNOLOGY June 2011 The author hereby grants MIT permission to reproduce and distribute publicly paper and electronic copies of this report document in whole or in part Copyright © Massachusetts Institute of Technology (MIT) All rights reserved Signature of Author: Department of Nuclear Science and Engineering Department of Mechanical Engineering March 31, 2011 Certified by: Michael J. Driscoll Professor Emeritus of Nuclear Science and Engineering Thesis Co-Supervisor Certified by: Charles W. Forsberg Executive Director, MIT Nuclear Fuel Cycle Project Thesis Co-Supervisor Certified by: Mark S. Welsh Professor of Practice of Naval Construction and Engineering Thesis Reader Accepted by: David E. Hardt Ralph E. and Eloise F. Cross Professor of Mechanical Engineering Chairman, Department Committee on Graduate Studies Accepted by: Mujid S. Kazimi TEPCO Professor of Nuclear Science and Engineering Chair, Department Committee on Graduate Students (This Page Intentionally Blank) 2 Nuclear Tanker Producing Liquid Fuels from Air and Water By Lieutenant John M. Galle-Bishop, United States Navy Submitted to the Department of Nuclear Science and Engineering and the Department of Mechanical Engineering in partial fulfillment for the requirements for the degrees of Master of Science in Nuclear Science and Engineering and Master of Science in Naval Architecture/Marine Engineering Abstract Emerging technologies in CO2 air capture, high temperature electrolysis, microchannel catalytic conversion, and Generation IV reactor plant systems have the potential to create a shipboard liquid fuel production system that will ease the burdened cost of supplying fuel to deployed naval ships and aircraft. Based upon historical data provided by the US Navy (USN), the tanker ship must supply 6,400 BBL/Day of fuel (JP-5) to accommodate the highest anticipated demand of a carrier strike group (CSG). Previous investigation suggested implementing shipboard a liquid fuel production system using commercially mature processes such as alkaline electrolysis, pressurized water reactors (PWRs), and methanol synthesis; however, more detailed analysis shows that such an approach is not practical. Although Fischer-Tropsch (FT) synthetic fuel production technology has traditionally been designed to accommodate large economies of scale, recent advances in modular, microchannel reactor (MCR) technology have to potential to facilitate a shipboard solution. Recent advances in high temperature co-electrolysis (HTCE) and high temperature steam electrolysis (HTSE) from solid oxide electrolytic cells (SOECs) have been even more promising. In addition to dramatically reducing the required equipment footprint, HTCE/HTSE produces the desired synthesis gas (syngas) feed at 75% of the power level required by conventional alkaline electrolysis (590 MWe vs. 789 MWe). After performing an assessment of various CO2 feedstock sources, atmospheric CO2 extraction using an air capture system appears the most promising option. However, it was determined that the current air capture system design requires improvement. In order to be feasible for shipboard use, it must be able to capture CO2 in a system only ¼ of the present size; and the current design must be modified to permit more effective operation in a humid, offshore environment. Although a PWR power plant is not the recommended option, it is feasible. Operating with a Rankine cycle, a PWR could power the recommended liquid fuel production plant with a 2,082 MWth reactor and 33% cycle efficiency. The recommended option uses a molten salt- cooled advanced high temperature reactor (AHTR) coupled to a supercritical carbon dioxide (S- o CO2) recompression cycle operating at 25.0 MPa and 670 C. This more advanced 1,456 MWth option has a 45% cycle efficiency, a 42% improvement over the PWR option. In terms of reactor power heat input to JP-5 combustion heat output, the AHTR is clearly superior to the PWR (31% vs. 22%). In order to be a viable concept, additional research and development is necessary to develop more compact CO2 capture systems, resolve SOEC degradation issues, and determine a suitable material for the molten salt/S-CO2 heat exchanger interface. Thesis Supervisors: Dr. Charles Forsberg, Prof. Michael Driscoll, Prof. Mark Welsh Thesis Title: Nuclear Tanker Producing Liquid Fuels from Air and Water 3 (This Page Intentionally Blank) 4 Acknowledgements In my opinion, what makes MIT special are its brilliant students and faculty. Attending class, researching ideas, and debating the merits of cutting edge engineering concepts with these wonderful people has been a tremendously rewarding privilege. I am extremely grateful for the patience, guidance, and insight my remarkable thesis advisors in the Nuclear Engineering Department, Dr. Charles Forsberg and Prof. Michael Driscoll, have provided me during the course of my research. I am equally grateful to my Naval Architecture and Marine Engineering advisor, Prof. Mark Welsh. His encouragement and assistance was essential in facilitating a thesis topic acceptable for two degrees. Although I have been fortunate to learn from many of my colleagues during my study, two of my friends deserve special recognition. First, I would like to extend my most sincere gratitude to my colleague, and good friend, Geoffrey Haratyk, for providing me with invaluable assistance in understanding the complicated concepts underpinning high temperature co-electrolysis (HTCE), as well as with assistance in describing the process in a MATLAB script. I also want to thank another good friend, Martin Kulhanek, for providing invaluable FORTRAN coding assistance, and for helping me reason through the various power cycle options. Several industry professionals also provided me invaluable insight and information. I want to thank RDML Thomas Moore, CAPT Brian Osgood, LCDR Stanley Dimirack, and MAJ Greg Johnson from Naval Sea Systems Command for providing me with the historical fuel consumption data I requested. I also appreciate the insights from Heather Willauer at the Naval Research Laboratory. Dr. Carl Stoots and Dr. James O’Brien of Idaho National Laboratory provided me crucial information regarding the reaction rate constant they empirically derived for their HTCE research, as well as for the space and weight characteristics of solid oxide electrolytic cell stacks. I want to thank Dr. Subhash Dutta for patiently explaining key aspects of the Fischer-Tropsch process within microchannel reactors, as well as providing me very useful performance and cost metrics. I am also pleased with the assistance and insights provided by Jeff McDaniels, Dr. Terry Mazanec, and Tad Dritz from Velocys regarding microchannel technology. Finally, I want to extend my deepest love and gratitude to my wonderful wife, Yvonne, for all of her encouragement, support, and love. 5 (This Page Intentionally Blank) 6 Table of Contents Abstract ......................................................................................................................................................... 3 Acknowledgements ....................................................................................................................................... 5 Tables .......................................................................................................................................................... 11 Figures ......................................................................................................................................................... 13 1 Introduction ........................................................................................................................................ 17 1.1 The Logistical Burden of Delivering Fuel ..................................................................................... 17 1.2 Synfuel Production Plant ............................................................................................................. 22 1.3 Previous Investigation ................................................................................................................. 24 1.4 A New Look ................................................................................................................................. 26 1.5 Thesis Outline .............................................................................................................................. 27 1.6 Chapter 1 References .................................................................................................................. 28 2 Fuel Requirements .............................................................................................................................. 31 2.1 The Case for a Single Fuel at Sea ................................................................................................. 31 2.2 Fuel Demand ............................................................................................................................... 33 2.2.1 F-76 Fuel Consumption ......................................................................................................
Details
-
File Typepdf
-
Upload Time-
-
Content LanguagesEnglish
-
Upload UserAnonymous/Not logged-in
-
File Pages313 Page
-
File Size-