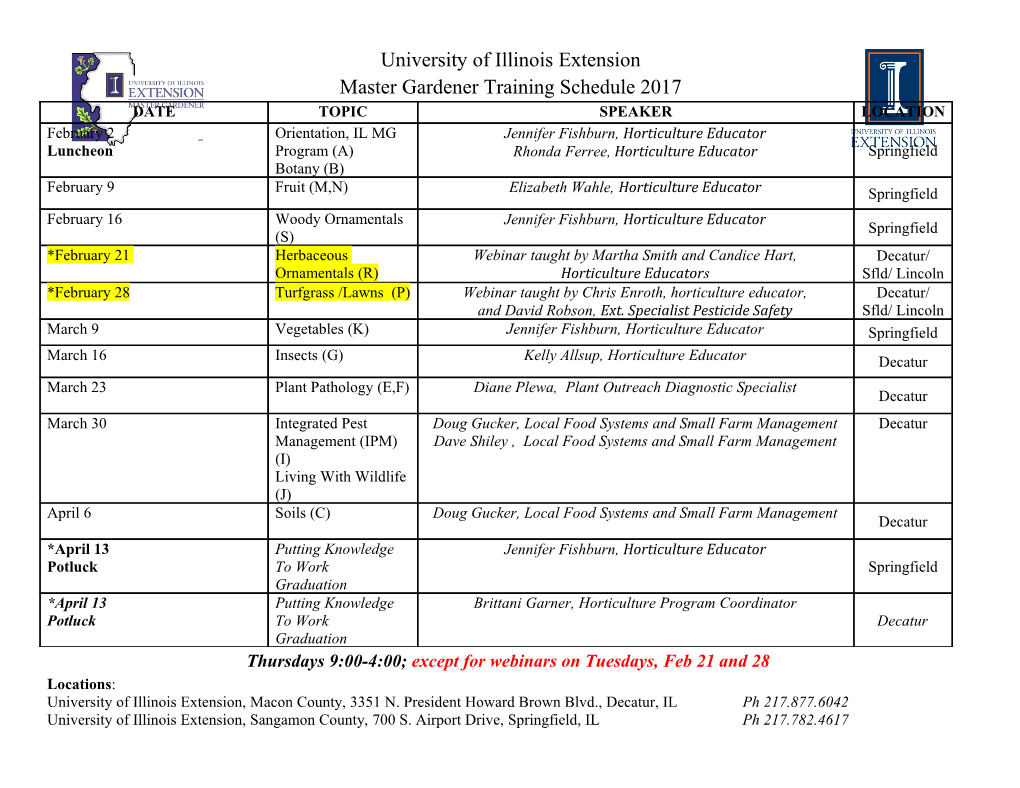
A NOVEL APPROACH TO DIAMONDLIKE CARBON BASED MID-INFRARED ATTENUATED TOTAL REFLECTANCE SPECTROELECTROCHEMISTRY A Thesis Presented to The Academic Faculty by Nicola Menegazzo In Partial Fulfillment of the Requirements for the Degree Doctor of Philosophy in Chemistry Georgia Institute of Technology May 2007 A NOVEL APPROACH TO DIAMONDLIKE CARBON BASED MID-INFRARED ATTENUATED TOTAL REFLECTANCE SPECTROELECTROCHEMISTRY Approved by: Dr. Boris Mizaikoff, Advisor Dr. Jiři Janata School of Chemistry and Biochemistry School of Chemistry and Biochemistry Georgia Institute of Technology Georgia Institute of Technology Dr. Lawrence Bottomley Dr. William Hunt School of Chemistry and Biochemistry School of Electrical and Computer Georgia Institute of Technology Engineering Georgia Institute of Technology Dr. Miroslawa Josowicz Date Approved: November 6, 2006 School of Chemistry and Biochemistry Georgia Institute of Technology Compton: "The Italian navigator has landed in the New World." Conant: "How were the natives?" Compton: "Very friendly." From Arthur Compton’s announcement to James Conant (chairman of the National Defense Research Committee) that Enrico Fermi had successfully achieved a self- sustained nuclear chain reaction on December 2nd, 1942. ACKNOWLEDGEMENTS Several people have been involved in the development of this work. I ask for the forgiveness of those individuals whose names are not mentioned here, as there would be too many for all to be included in the space allotted. I would like to thank Dr. Boris Mizaikoff and Dr. Christine Kranz for giving me the opportunity of working with them over the span of my graduate studies. Together, they provided a highly creative environment allowing nearly unrestricted exploration of countless topics, interests and ideas. I’m equally indebted to both past and present members of the Applied Sensors Laboratory for sharing their knowledge in the lab, and companionship outside. Dr. Jiři Janata, Dr. Miroslawa Josowicz, Dr. Lawrence Bottomley and Dr. William Hunt are gratefully acknowledged for serving as committee members and for scientific discussions undertaken on numerous occasions. In addition, I would like to thank the Thin Film Technology group at the Laser Center Leoben headed by Dr. Wolfgang Waldhauser, as well as Dr. Roger J. Narayan and Dr. Chunming Jin at the University of North Carolina (Chapel Hill) for collaborating in the DLC portion of this work. Financial support from the ExxonMobil Research and Engineering Company in Annandale (NJ) is also acknowledged. Finally, all of this would not have been possible without the unconditional support I received from my family throughout all my studies. Thank you! iv TABLE OF CONTENTS ACKNOWLEDGEMENTS iv LIST OF TABLES viii LIST OF FIGURES ix LIST OF ABBREVIATIONS xiii SUMMARY xvi 1 INTRODUCTION 1 1.1 Spectroelectrochemistry 2 1.1.1 External reflection spectroelectrochemistry 7 1.1.1.1 Electrochemically modulated infrared reflection spectroscopy (EMIRS) 7 1.1.1.2 Subtractively normalized interfacial Fourier transform infrared spectroscopy (SNIFTIRS) 8 1.1.1.3 Polarization modulation infrared reflection absorption spectroscopy (PM-IRRAS) 10 1.1.2 Transmission spectroelectrochemistry 11 1.2 References 13 2 BACKGROUND 17 2.1 Attenuated total reflectance spectroscopy 17 2.1.1 Principles of ATR 17 2.1.2 Spectroelectrochemistry with mid-IR ATR 21 2.2 Diamondlike carbon 25 2.2.1 Introduction 25 2.2.2 Pulsed laser deposition (PLD) of DLC 28 2.2.3 Electronic properties 29 2.2.4 Electrochemical characterization of conducting DLC 32 2.2.5 Imaging with scanning electrochemical microscopy 38 2.3 Redox polymer membranes 39 2.3.1 Introduction 39 2.3.2 Redox polymers in mid-IR spectroelectrochemistry 42 2.4 References 43 3 CHARACTERIZATION OF NITROGEN-DOPED DIAMONDLIKE CARBON MATERIALS 54 3.1 Motivation 54 3.1.1 Nitrogen-doped diamondlike carbon 55 3.2 Experimental 57 v 3.2.1 Pulsed laser deposition system 57 3.2.2 Physical characterization 58 3.2.3 Electrochemical characterization 59 3.3 Results 62 3.3.1 X-ray photoelectron spectroscopy studies 62 3.3.2 Raman spectroscopic studies 65 3.3.3 Mid-infrared spectroscopic studies 68 3.3.4 Electrochemical studies 69 3.3.5 Spectroelectrochemical studies 76 3.4 Final remarks 82 3.5 References 84 4 CHARACTERIZATION OF NOBLE METAL-DIAMONDLIKE CARBON NANOCOMPOSITE MATERIALS 92 4.1 Motivation 92 4.1.1 Metal-diamondlike carbon (DLC) nanocomposites 92 4.2 Experimental 94 4.2.1 Pulsed laser deposition system 94 4.2.2 Physical characterization 95 4.2.3 Electrochemical characterization 97 4.3 Results 97 4.3.1 Rutherford backscattering spectroscopy 98 4.3.2 X-ray photoelectron spectroscopy studies 100 4.3.3 Raman spectroscopic studies 104 4.3.4 Transmission electron, scanning electron and atomic force microscopy 105 4.3.5 Mid-infrared spectroscopic studies 107 4.3.6 Electrochemical studies 108 4.3.7 Scanning electrochemical microscopy studies 111 4.3.8 Spectroelectrochemical studies 112 4.4 Final remarks 115 4.5 References 117 5 SPECTROSCOPIC AND ELECTROCHEMICAL STUDIES ON THE DEPOSITION CONDITIONS FOR CATHODICALLY ELECTROPOLYMERIZED POLY(4-VINYLPYRIDINE) IN ACETONITRILE 123 5.1 Motivation 123 5.1.1 Poly(4-vinylpyridine) as an anion selective membrane 125 5.2 Experimental 126 5.2.1 Spectroscopic characterization 126 5.2.2 Polymer formation and electrochemical characterization 127 vi 5.3 Results 128 5.3.1 Infrared spectroscopic studies 128 5.3.2 Ultraviolet spectroscopic studies 130 5.3.3 1H Nuclear magnetic resonance spectroscopic studies 132 5.3.4 Electrochemical characterization 136 5.4 Final remarks 145 5.5 References 146 6 CONCLUSION AND OUTLOOK 151 6.1 Nitrogen-doped DLC 151 6.2 Metal-DLC nanocomposite 152 6.3 Electropolymerization of poly(4-VP) 153 vii LIST OF TABLES Table 2.1: Penetration depths of common IR transparent materials in contact with water (n2 = 1.33, θ = 45 º) 20 Table 3.1: Measured thicknesses of N-DLC layers determined by stylus profilometry. 58 Table 3.2: Compositional analysis of the N-DLC layers (n = 3). 64 3+/2+ 3-/4- Table 3.3: Peak separation for the Ru(NH3)6 and Fe(CN)6 redox couples at (N- )DLC layers at 0.1 V/s (n = 3). 69 3+/2+ 3-/4- Table 3.4: Kinetic parameters for the Ru(NH3)6 and Fe(CN)6 redox couples for untreated DLC layers at 0.1 V/s (n = 3). 70 3+/2+ 3-/4- Table 3.5: Peak separation of Ru(NH3)6 and Fe(CN)6 redox couples for cathodically treated (N-)DLC layers at 0.1 V/s (n = 3). 73 3+/2+ 3-/4- Table 3.6: Kinetic parameters for the Ru(NH3)6 and Fe(CN)6 redox couples for cathodically treated (N-)DLC layers at 0.1 V/s (n = 3). 74 Table 3.7: Comparison of ks values obtained with N-DLC electrodes in this study with those published for several carbon electrodes in literature. 74 Table 3.8: Spectral assignment of the absorption bands in Figure 3.11(a). 79 Table 4.1: Thickness of the metal-DLC nanocomposite layers determined by stylus profilometry. 95 Table 4.2: Metal content in the DLC nanocomposite layers determined by RBS 98 Table 4.3: Compositional analysis of the metal-DLC nanocomposite layers presented in this study. 103 3+/2+ 3-/4- Table 4.4: Peak separation of Ru(NH3)6 and Fe(CN)6 redox species with respect to the metal concentration at 0.1 V/s (n = 3). 110 Table 4.5: Kinetic parameters for the metal-DLC layers derived from simulations (n = 3). 111 viii LIST OF FIGURES Figure 2.1: Schematic representation of the TIR principle. 19 Figure 2.2: Schematic for spectroelectrochemical analysis in (a) transmission and (b) external reflection mode. 22 Figure 2.3: Ternary phase diagram displaying the possible compositions of diamondlike carbon layers (reproduced from Robertson39). 26 Figure 2.4: Schematic representation of (a) pulsed laser deposition chamber, and (b) metal-graphite hybrid target used for the deposition of nanocomposite materials (Chapter 4). 29 Figure 2.5: Correlation between the sp2-hybridized carbon content and the optical gap displayed by different DLC layers (reproduced from Robertson67). 30 Figure 2.6: Graphic representation of electronic defects commonly encountered in DLC layers due to (a) “dangling bonds”, and (b) odd π electron orbitals. 31 Figure 2.7: (a) Triangular waveform applied during cyclic voltammetry, and (b) cyclic voltammogram obtained from a fast one-electron transfer reaction. 33 Figure 2.8: Current response measured at the ultramicroelectrode for (a) negative, and (b) positive feedback mode. 39 Figure 2.9: Examples of redox polymers with (a) covalently, and (b) electrostatically bonded redox sites. 40 Figure 2.10: Mechanism for the electropolymerization of 2-vinylpyridine.113 41 Figure 3.1: (a) Top and (b) side view of the custom-built Teflon cell utilized in the electrochemical characterization of DLC. 60 Figure 3.2: (a) Side and (b) top view schemes of the PEEK spectroelectrochemical cell. Optical micrographs of (c) the PEEK cover plate, and (d) the fully assembled spectroelectrochemical setup on the optical mirror bench. 62 Figure 3.3: (a) X-ray photoelectron spectra of (i) N-DLC (sample N #2) and (ii) non- doped DLC. (b) High resolution C 1s photoemission peak doped and non- doped DLC, and (c) high resolution N 1s peak of N-DLC . 64 Figure 3.4: Anticipated changes in the Raman spectra of carbon films with increasing disorder and sp3-hybridized carbon content. 66 Figure 3.5: Visible Raman spectra of non-doped and nitrogen-doped DLC layers. 67 Figure 3.6: Changes in (a) ID/IG and (b) FWHM of the G-band with respect to the nitrogen content (n = 3). 68 Figure 3.7: (a) Single beam spectra of (i) bare ZnSe, (ii) N-DLC coated ZnSe, and (iii) non-doped DLC coated ZnSe.
Details
-
File Typepdf
-
Upload Time-
-
Content LanguagesEnglish
-
Upload UserAnonymous/Not logged-in
-
File Pages172 Page
-
File Size-