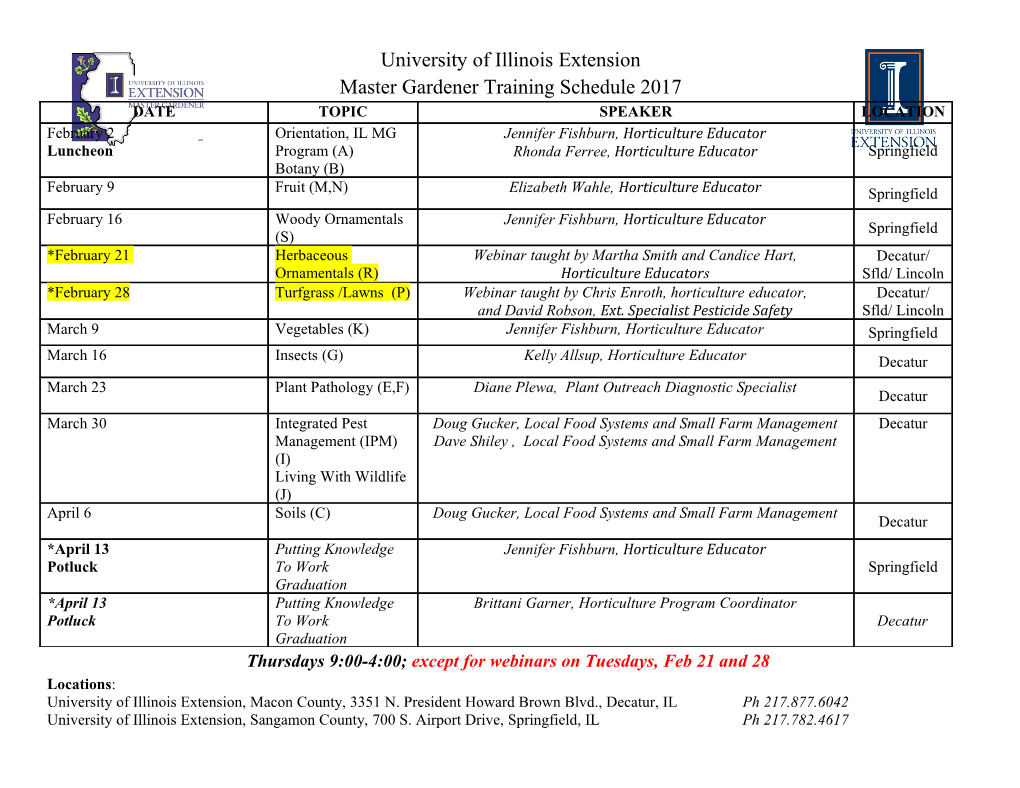
TECHNISCHE UNIVERSITÄT MÜNCHEN Fakultät für Maschinenwesen Lehrstuhl für Luftfahrtsysteme Multidisciplinary Design of Aeronautical Composite Cycle Engines Sascha Kaiser Vollständiger Abdruck der von der Fakultät für Maschinenwesen der Technischen Universität München zur Erlangung des akademischen Grades eines Doktor-Ingenieurs (Dr.-Ing.) genehmigten Dissertation. Vorsitzender: Prof. Dr.-Ing. Volker Gümmer Prüfer der Dissertation: 1. Prof. Dr.-Ing. Mirko Hornung 2. Adjunct Prof. Dr. Anders Lundbladh Chalmers University of Technology, Gothenburg, Sweden Die Dissertation wurde am 28.02.2019 bei der Technischen Universität München eingereicht und durch die Fakultät für Maschinenwesen am 28.10.2019 angenommen. i Acknowledgement We set out to achieve the lowest sfc in the world, regardless of weight and bulk; so far we’ve achieved the weight and the bulk. – Frank Owner about the Bristol Proteus turboprop engine This thesis is the result of six years of work. It would not have been possible without the support from many people, who cannot be counted or named individually. To some of them I want to convey my gratitude on this page. First, I want to thank my thesis supervisor Prof. Mirko Hornung and my second examiner Prof. Anders Lundbladh for their continuous support throughout the thesis in guiding the research topics into the right direction and asking the right questions at the right time. Most of the work has been conducted at the research institute Bauhaus Luftfahrt e.V., where a motivating and liberal working environment allowed me to explore many different perspectives of my work before converging on the research question. I want to thank my dear colleagues, who held up the good spirit at all times, and fostered a supportive research environment. In particular, I want to thank Oliver Schmitz and Clement Pornet, who accompanied my early years in research, Patrick Vratny, Michael Schmidt, Ulrich Kling, Philipp Heinemann and Anne Schuster, who became outstanding friends, and the core propulsion group around Arne Seitz and Markus Nickl. During my work, I could rely on profound and thought provoking feedback from colleagues in industry. Stefan Donnerhack was a strong promoter of my work, and enabled research partnerships that will most likely persist beyond this thesis. Hermann Klingels is the ingeni- ous inventor behind the concept with a matchless intuition for engineering solutions. Jens Trübenbach and Alyssa Butler accompanied the finishing. Last but not least, I could not have accomplished such an enduring task without a solid basis. My family and my parents gave me their absolute support and backing, and never missed to put a smile on my face, when I needed it. Thank you Sonja, Sophie and Alexander. Sascha Kaiser Munich, 29th January 2020 ii Preamble The method development for this thesis was partially conducted in the projects LEMCOTEC (Low Emissions Core-Engine Technologies) and ULTIMATE (Ultra Low emission Technology Innovations for Mid-century Aircraft Turbine Engines). LEMCOTEC was co-funded by the European Commission within the Seventh Framework Programme (2007-2013) under the Grant Agreement n° 283216. ULTIMATE received funding from the European Union’s Ho- rizon 2020 research and innovation programme under grant agreement n° 633436. iii Abstract The efficiency of aero engines improved with every new generation. Improvements ofturbo components, as well as increase of overall pressure ratio and combustor temperatures raised thermal efficiency, while higher bypass ratio fans raised propulsive efficiency. With thenew- est generation of turbofans, the improvement potential of the Joule-/Brayton-cycle and the propulsive efficiency approach technically viable limits. At the same time, ambitious emis- sion reduction targets require further leaps in engine efficiency. The Composite Cycle Engine concept introduced in this thesis is a candidate for a step change in core engine architectures. The concept introduces a piston engine to the high-pressure part of a turbofan core engine, where it drives a high pressure compressor. Piston engines burn fuel at constant volume, which brings along a free pressure rise. They enable higher cycle temperatures and pressures due to instationary operation. The created efficiency improvement comes at the price of higher weight, size and pressure oscillations. This thesis answers the question, if the resulting fuel burn improvement can achieve future emission reduction targets with detailed multidiscip- linary component modelling. The piston engine is simulated with a 0D time-resolved model. Engine weight and size are estimated on component-level to generate physically adequate results for comparison with a turbofan. A sophisticated 2-zone NOx emissions model was developed to evaluate compliance with projected certification targets. The appraisal of the technical viability was supported by detailed preliminary, conceptual design of the core engine. Particularly piston engine features were specified such as oper- ating mode, layout, lubrication, cooling and coupling to turbo components. The choice of component limits was supported by data from production piston engines from all fields of applications. The concept was evaluated on a short-to-medium range aircraft platform with a year 2035 technological level. The engine was evaluated against a projected geared turbofan. With a four-stroke piston engine, overall efficiency can be increased by 12.3 %. Mission fuel burn reduces only by 5.7 % as engine mass increases significantly from 3 502 kg to 5 962 kg. NOx emissions triple. Using a two-stroke piston engine almost allows to meet fuel burn reduction targets for the year 2035 with a further 1.1 % improvement due to lower engine weight, although efficiency is worse. The additional use of an intercooler can alleviate thermal and NOx problems, but does not provide better efficiency or engine mass. A more advanced free-piston engine concept could considerably improve engine efficiency and mass. The main challenges identified are high mechanical and thermal loads on the piston engines. v Contents Vorwort i Abstract iii List of Figures xii List of Tables xiv Nomenclature xv 1 Introduction1 2 The Composite Cycle Engine Concept3 2.1 Thermodynamic Benefits of Composite Cycle Engines.............3 2.2 Piston Engines in Aviation.............................6 2.3 Experience and Research on the Composite Cycle Engine Architecture.... 11 2.4 Alternative Cycles and Closed Volume Combustion Concepts......... 15 3 Methods 19 3.1 Fluid Properties.................................. 19 3.2 Propulsion System Simulation........................... 23 3.3 Piston System Simulation............................. 29 3.3.1 Heat Losses................................. 34 3.3.2 Numerical Integration........................... 36 3.3.3 Implementation into Engine Simulation Environment.......... 37 3.3.4 Validation.................................. 39 3.3.5 Mechanical Losses............................. 42 3.4 Heat Exchanger Modelling............................. 45 vi Contents 3.5 Flow Path Generation............................... 47 3.6 Mass Estimation.................................. 48 3.7 Aircraft Level Assessment............................. 53 3.8 Instationary Operation............................... 54 3.8.1 Impact of Pulsating Flow on Turbo Components............ 54 3.8.2 Mechanical Oscillation........................... 56 3.9 Emissions...................................... 57 3.9.1 CO2 Emissions............................... 57 3.9.2 NOx Emissions............................... 58 3.9.3 Noise Emissions.............................. 67 3.9.4 Other Emissions and Interference between Emission Targets...... 68 4 Conceptual Design of Composite Cycle Engines 71 4.1 Evaluation Platform................................ 71 4.2 Overall Engine Design............................... 77 4.3 Piston Engine Design................................ 81 4.4 Synergistic Technologies.............................. 87 5 Results of Selected Composite Cycle Engine Concepts 91 5.1 Baseline Four-Stroke Engine Concept....................... 91 5.1.1 Flow Path and Mass............................ 95 5.1.2 Fuel Burn.................................. 98 5.1.3 Part-Load Behaviour............................ 99 5.1.4 NOx Emissions............................... 100 5.1.5 Technology Sensitivity Study....................... 101 5.2 Alternative Piston Engine Concepts....................... 102 5.2.1 Two-Stroke Piston Engine......................... 102 5.2.2 Intercooled Composite Cycle Engine................... 105 5.2.3 Free-Piston Engine............................. 108 5.2.4 Summary.................................. 111 5.3 Technology Road Mapping............................. 116 6 Conclusion and Outlook 119 Bibliography 123 Contents vii A Appendix 143 A.1 Turbo Component Setup.............................. 143 A.2 Validation 2-Stroke Engine Neural Network................... 144 A.3 Supplementary figures............................... 145 A.4 Supplementary Data................................ 147 ix List of Figures 1.1 Engine fuel burn reduction targets by engine manufacturers in the context of SRIA and NASA N+3 targets...........................1 2.1 Temperature 푇 over specific entropy 푠 diagram of the Composite Cycle in contrast to the Joule-/Brayton-cycle at ToC conditions (adapted from [17]). Station nomenclature according to Figure 3.2. Station 41 after addition of turbine stator cooling air, station 44 after turbine work extraction.......4 2.2
Details
-
File Typepdf
-
Upload Time-
-
Content LanguagesEnglish
-
Upload UserAnonymous/Not logged-in
-
File Pages178 Page
-
File Size-