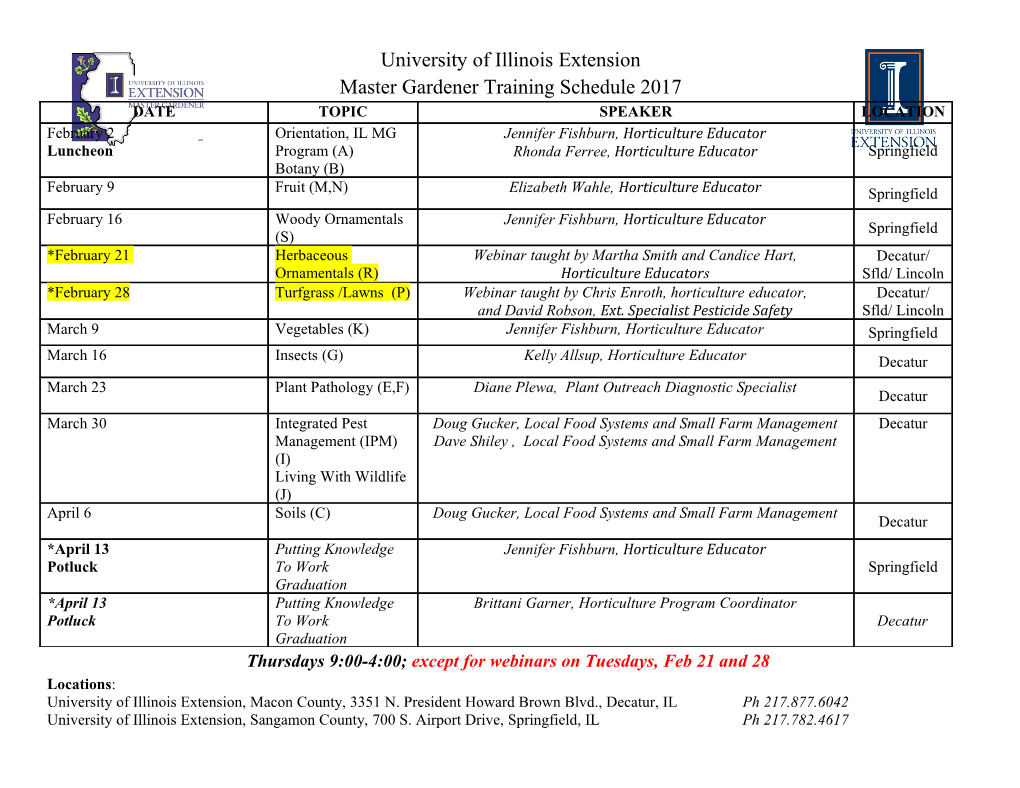
Ionization rates in a Bose-Einstein condensate of metastable Helium Olivier Sirjean, Signe Seidelin, José Carlos Viana Gomes, Denis Boiron, Christoph I Westbrook, Alain Aspect, G. V. Shlyapnikov To cite this version: Olivier Sirjean, Signe Seidelin, José Carlos Viana Gomes, Denis Boiron, Christoph I Westbrook, et al.. Ionization rates in a Bose-Einstein condensate of metastable Helium. Physical Review Letters, American Physical Society, 2002, 89, pp.220406. 10.1103/PhysRevLett.89.220406. hal-00118659 HAL Id: hal-00118659 https://hal.archives-ouvertes.fr/hal-00118659 Submitted on 14 Apr 2016 HAL is a multi-disciplinary open access L’archive ouverte pluridisciplinaire HAL, est archive for the deposit and dissemination of sci- destinée au dépôt et à la diffusion de documents entific research documents, whether they are pub- scientifiques de niveau recherche, publiés ou non, lished or not. The documents may come from émanant des établissements d’enseignement et de teaching and research institutions in France or recherche français ou étrangers, des laboratoires abroad, or from public or private research centers. publics ou privés. VOLUME 89, NUMBER 22 P H Y S I C A L R E V I E W L E T T E R S 25 NOVEMBER 2002 Ionization Rates in a Bose-Einstein Condensate of Metastable Helium O. Sirjean, S. Seidelin, J. Viana Gomes,* D. Boiron, C. I. Westbrook, and A. Aspect Laboratoire Charles Fabry de l’Institut d’Optique, UMR 8501 du CNRS, F-91403 Orsay Cedex, France G.V. Shlyapnikov FOM Institute for Atomic and Molecular Physics, Kruislaan 407, 1098 SJ Amsterdam, The Netherlands and Russian Research Center Kurchatov Institute, Kurchatov Square, 123182 Moscow, Russia (Received 5 August 2002; published 12 November 2002) We have studied ionizing collisions in a BEC of metastable He. Measurements of the ion production rate combined with measurements of the density and number of atoms for the same sample allow us to estimate both the two- and three-body contributions to this rate. A comparison with the decay of the atom number indicates that ionizing collisions are largely or wholly responsible for the loss. Quantum depletion makes a substantial correction to the three-body rate constant. DOI: 10.1103/PhysRevLett.89.220406 PACS numbers: 34.50.–s, 05.30.–d, 67.65.+z, 82.20.Pm The observation of Bose-Einstein condensation (BEC) decay rates in He*. The dominant two-body decay 3 of metastable helium (He in the 2 S1 state, denoted He*) mechanisms, called Penning ionization, [1,2] constituted a pleasant surprise for experimentalists He He 1Seÿ although the possibility had been predicted theoretically He He ! (1) ÿ [3]. Success hinged, among other things, on a strong He2 e suppression of Penning ionization in the spin-polarized, are known to be suppressed by at least 3 orders of magni- magnetically trapped gas. Too high a rate of ionization tude in a spin-polarized sample, but the total rate con- would have prevented the accumulation of sufficient den- stant has not yet been measured. The three-body reaction, sity to achieve evaporative cooling. The ionization rate is not completely suppressed however, and when the atomic He He He ! He2 He 1mK density gets high enough, a magnetically trapped sample ,! He He 1Seÿ (2) of He* does produce a detectable flux of ions. As shown in [1], this signal can even be used as a signature of proceeds via three-body recombination followed by auto- BEC. The observation of ions from the condensate opens ionization of the excited molecule. Both reactions yield the possibility of monitoring in real time the growth one positive ion which is easily detected. We define colli- kinetics of a condensate [4]. This is an exciting prospect, sion rate constants according to the density loss in a dn n 2 3 but to quantitatively interpret the ion rate, one needs the thermal cloud: dt ÿ ÿ n ÿ Ln with n the local contributions of two- and three-body collisions. density, the (background gas limited) lifetime of the In this paper we use the unique features of metastable sample, and and L the two-body and three-body ioni- atoms to detect, in a single realization, the ionization zation rate constants defined for a thermal cloud [15]. The rate, the density, and the atom number. This allows us theoretical estimates of the rate constants at 1 K are ÿ14 3 ÿ1 ÿ26 6 ÿ1 to extract two- and three-body rate constants without 2 10 cm s [3,12] and L 10 cm s relying on fits to nonexponential decay of the atom num- [16], and the experimental upper limits were [1,2] ÿ14 3 ÿ1 ÿ26 6 ÿ1 ber, which require good experimental reproducibility [5– 8:4 10 cm s and L 1:7 10 cm s . 7] and are difficult to interpret quantitatively [5]. After For a pure BEC, in the Thomas-Fermi regime with a estimating the ionization rate constants, a comparison number of atoms N0, and a peak density n0, one can with the decay of the atom number reveals no evidence calculate the expected ionization rate per trapped atom: for collisional avalanche processes. Thus, by contrast with ion rate 1 2 8 87 2 Rb [8], He* seems to be a good candidate for studying ÿ 2 n0 3Ln : (3) N 0 7 63 0 the ‘‘hydrodynamic’’ regime [9], as well as the effects of 0 quantum depletion, i.e., a departure from the Gross- The numerical factors come from the integration over the Pitaevskii wave function in the Bogoliubov theory, due parabolic spatial profile and the fact that although two or to atomic interactions [10]. Indeed in our analysis of the three atoms are lost in each type of collision, only one ion three-body ionization process, quantum depletion makes is produced. The effective lifetime 0 is due to ioniz- a substantial correction [11]. ing collisions with the background gas. The factors i Much theoretical [3,12] and experimental [1,2,13,14] take into account the fact that the two- and three-particle work has already been devoted to estimating inelastic local correlation functions are smaller than those of a 220406-1 0031-9007=02=89(22)=220406(4)$20.00 2002 The American Physical Society 220406-1 VOLUME 89, NUMBER 22 P H Y S I C A L R E V I E W L E T T E R S 25 NOVEMBER 2002 thermal cloud. For a dilute BEC 2 1=2! and 3 1=3! thermal fraction we can distinguish is about 20%, with a [7,11]. Because the He*p scattering length (a) is so large, temperature on the order of the chemical potential. Runs 3 quantum depletion ( n0a leads to significant correc- with visible thermal wings were discarded. tions [11] to the ’s as we discuss below. To acquire the TOF signals corresponding to a given ion Much of our setup has been described previously rate, we turn off the rf shield, wait 50 ms, and then turn [1,17,18]. Briefly, we trap up to 2 108 atoms at 1 mK off the magnetic trap and switch the MCP to analog in a Ioffe-Pritchard trap with a lifetime () of 90 s.We use mode. To be sure that the rf has no influence on the ion a ‘‘cloverleaf’’ configuration [19] with a bias field B0 rate, we use only the number of ions observed during the 150 mG. The axial and radial oscillation frequencies in 50 ms delay to get the rate. We fit the TOF signals to an the harmonic trapping potential are k 47 3Hzand inverted parabola squared as expected for a pure BEC in 2 1=3 ? 1800 50 Hz, respectively [!=2 k? the Thomas-Fermi regime and for a TOF width ( 5ms) 534 Hz]. A crucial feature of our setup is the detection narrow compared to the mean arrival time (100 ms) [1]. scheme, based on a two stage, single anode microchannel Under these assumptions, the chemical potential de- plate detector (MCP) placed below the trapping region. pends only on the TOF width, the atomic mass, and the Two grids above the MCP allow us either to repel positive acceleration of gravity [22], and thus can be measured ions and detect only the He* atoms, or to attract and quite accurately. Figure 1 shows that varies as expected 2=5 detect positive ions produced in the trapped cloud. as Nd with Nd the number of detected atoms in the To detect the ion flux, the MCP is used in counting quasipure BEC. A fit on a log-log plot gives a slope of mode: the anode pulses from each ion are amplified, 0.39. Residuals from the linear fit do not show any system- discriminated with a 600 ns dead time and processed by atic variation which is a good indication of the detection a counter which records the time delay between succes- linearity and of the proportionality between Nd and N0. sive events. Typical count rates around BEC transition are To determine the collision rate constants and L,we between 102 and 105 sÿ1. We have checked that the cor- need an absolute calibration of the number of atoms and relation function of the count rate is flat, indicating that the density. As discussed in Ref. [1], all the atoms are not there is no double counting nor any significant time detected, and the direct calibration has a 50% uncertainty correlation in the ion production. The dark count rate is which is responsible for the large uncertainty in the of order 1sÿ1. By changing the sign of the grid voltage, scattering length a.
Details
-
File Typepdf
-
Upload Time-
-
Content LanguagesEnglish
-
Upload UserAnonymous/Not logged-in
-
File Pages5 Page
-
File Size-