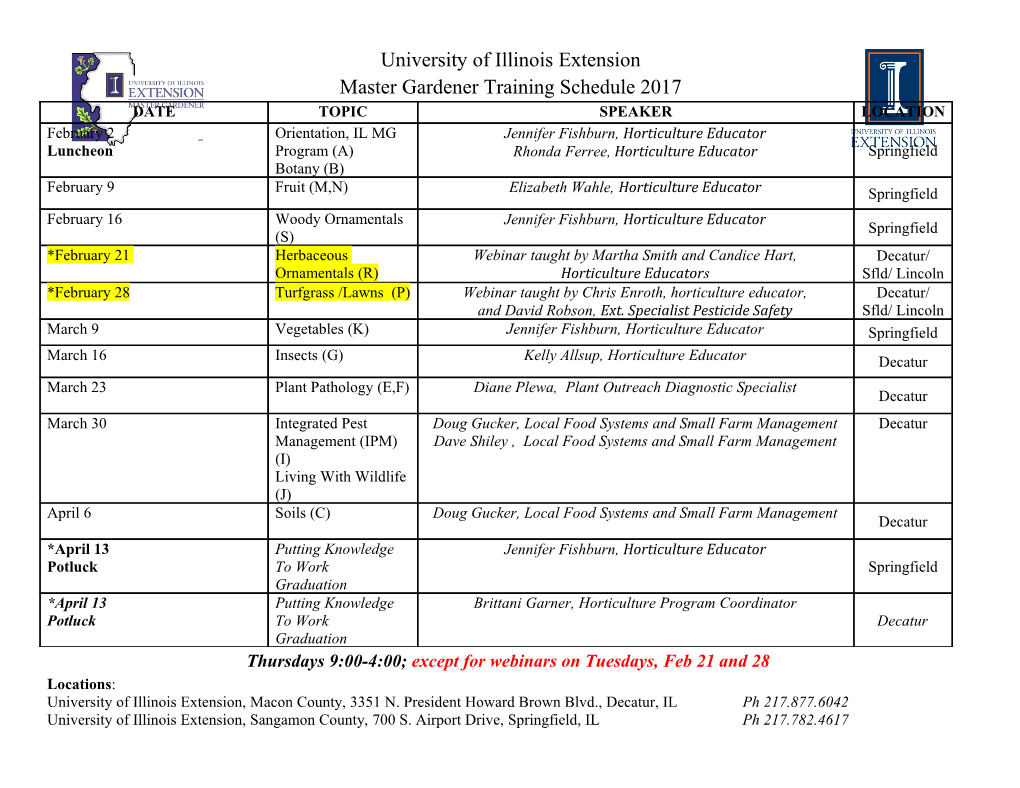
Calculation of rates for radioactive isotope beam production at TRIUMF by Fatima H. Garcia B.Sc., University of Calgary, 2013 Thesis Submitted in Partial Fulfillment of the Requirements for the Degree of Master of Science in the Department of Chemistry Faculty of Science c Fatima H. Garcia 2016 SIMON FRASER UNIVERSITY Fall 2016 All rights reserved. However, in accordance with the Copyright Act of Canada, this work may be reproduced without authorization under the conditions for “Fair Dealing.” Therefore, limited reproduction of this work for the purposes of private study, research, criticism, review and news reporting is likely to be in accordance with the law, particularly if cited appropriately. Approval Name: Fatima H. Garcia Degree: Master of Science (Chemistry) Title: Calculation of rates for radioactive isotope beam production at TRIUMF Examining Committee: Chair: Dr. Charles J. Walsby Associate Professor Dr. Corina Andreoiu Senior Supervisor Associate Professor Dr. Peter Kunz Co-Supervisor Adjunct Professor Dr. Daniel B. Leznoff Supervisor Professor Dr. Anne J. Trudel Supervisor Chief Safety Officer TRIUMF Dr. John D’Auria Internal Examiner Professor Emeritus Date Defended/Approved: November 15th, 2016 ii Abstract Access to new and rare radioactive isotopes is imperative for establishing fundamental knowledge and for its application in nuclear science. Rare Isotope Beam (RIB) facilities around the world, such as TRIUMF, work towards development of new target materials to generate increasingly exotic species, which are used in nuclear medicine, astrophysics and fundamental physics studies. At Simon Fraser University and TRIUMF, a computer simulation of the RIB targets used at the Isotope Separation and ACceleration (ISAC) facility of TRIUMF was built, to compliment existing knowledge and to support new tar- get material development. The simulation was built using the GEANT4 nuclear transport toolkit, and can simulate the production rate of isotopes from user-defined beam and tar- get characteristics. The simulation models the bombardment of a production target by an incident high-energy particle beam and calculates isotope production rates via fission, fragmentation and spallation. In-target production rates from the simulation were analysed and compared to production mechanisms within the simulation environment, other nuclear transport algorithms and to the experimentally measured yield rates from the ISAC yield station. Additionally, preliminary studies were conducted using these in-target production rates as illustrative examples, showing the capabilities and power of the simulation. Keywords: GEANT4; Radioactive Isotope Beams; Monte Carlo Simulations; TRIUMF- ISAC. iii Acknowledgements Completion of this work would have not been possible without the presence of some very important people. I would like to take this opportunity to acknowledge and thank them for their unwavering support. Without it, the document before you would not exist. To my mentor, Dr. Jason Donev, thank you for being a constant source of encourage- ment. Your stories and advice have helped me navigate through the academic maze. To my supervisors, Dr. Corina Andreoiu and Dr. Peter Kunz, thank you for the op- portunity to work on this project and for the constant support during its completion. Your expertise has been invaluable and your lessons essential to my success. To the members of my committee, Dr. Daniel Leznoff and Dr. Anne Trudel, thank for your insight and presence. The support I’ve received from you has been integral to this work. To current and former members of the SFU Nuclear Science groups, specially Jennifer Pore, thank you. For answering all my silly questions, helping me through the struggles and teaching me about how to survive and thrive in graduate school. I am very fortunate to have shared an office with such a brilliant group of people. To my friends at SFU and at home, particularly Yumeela and Allison, thank you. For all the early mornings, late nights, agonizing times, and hilarious moments. They, and you, have been an essential and amazing and fundamental part of my graduate school experience. Finally, to my parents, Vilma and Roberto Garcia and my sister Lourdes Garcia, thank you. For everything. For your unyielding support, tireless encouragement, and uncon- ditional love. Your sacrifices, wisdom and example have been an unfaltering source of inspiration and motivation throughout my entire life. I know that, without you, I could not have made it this far. Los quiero mucho. iv Table of Contents Approval ii Abstract iii Acknowledgements iv Table of Contents v List of Tables viii List of Figures ix List of Abbreviations xi 1 Introduction 1 1.1 Nuclear Theory . 2 1.1.1 The Nucleus . 2 1.1.2 The Nuclide Chart . 3 1.1.3 Nuclear Reactions . 5 1.1.4 Nuclear Decay . 8 1.2 TRIUMF and ISAC . 13 1.2.1 ISOL Technique . 13 1.2.2 ISAC at TRIUMF . 14 1.2.3 ISAC Yield Station . 18 1.3 GEANT4 . 22 1.3.1 Framework . 22 1.3.2 Geometry . 24 1.3.3 Physics Lists . 25 2 Simulation 30 2.1 Simple Geometry . 30 2.1.1 Primary particle description . 30 2.1.2 Standard Target Description . 31 v 2.1.3 Detection Mechanisms . 32 2.2 Data Output & Processing . 33 2.3 Error Analysis . 34 2.4 Benchmark Products . 34 2.5 Competing Models . 35 2.5.1 Silberberg-Tsao . 35 2.5.2 FLUKA . 35 2.5.3 IAEA Benchmark of Spallation Models . 36 2.6 Extended Geometry . 36 2.7 Current Simulation Status . 38 3 Results 39 3.1 Physics Lists Results . 39 3.2 In-target Production Values . 42 3.2.1 Results Summary . 51 4 Further Studies 52 4.1 Cross Section Verification . 52 4.1.1 Bertini & Binary Cascades . 53 4.1.2 Liège Cascade . 53 4.1.3 Validation conclusion . 53 4.2 Energy Deposition . 56 4.3 Release Times and Release Efficiencies . 57 4.4 Thorium Oxide Target Runs . 61 4.4.1 Medical Isotope Production . 63 4.5 Alternative targets . 64 4.6 Incident Particle Energy Dependence . 65 4.7 Kinetic Energy Distributions . 68 4.8 In-target decay . 71 4.9 Summary . 74 5 Future Work & Conclusions 75 5.1 Outlook . 75 5.2 Summary . 76 Bibliography 78 Appendix A Errors 84 Appendix B Alkaline Earth Metals 88 vi Appendix C Liège cascade cross section validation 92 Appendix D Target Materials 96 vii List of Tables Table 2.1 The materials implemented in the GEANT4 simulation include all ma- terials previously used at TRIUMF, along with a selection of other materials. 32 Table 4.1 Energy deposition values returned from GEANT4 simulation and SRIM software [70], using the standard target disk configuration. 57 Table 4.2 Half lives of francium isotopes between 211Fr and 214Fr, given in sec- onds. Values taken from NNDC [12]. 58 Table 4.3 Release parameters for the three intra-nuclear cascades used in the simulation. 60 Table 4.4 In-target production rates of medical isotopes produced by a thorium target and compared to a uranium target, given in particles per second and normalized to reflect 1 mA of proton current through the target. 64 Table 4.5 Materials implemented into the simulation. Target materials are either analogous to target materials used at ISAC or they have been imple- mented as hypothetical targets. Lead and aluminium targets have thickness of 87.3 and 12.1 mg/cm2 respectively, while all other targets have a thickness of 0.05 mol/cm2, and a target length of 5 cm. 65 Table 4.6 Stopping ranges for rubidium isotopes with kinetic energy between 30 MeV and 150 MeV, as calculated by the SRIM Software [70], with a target composed of 238U with a density of 2.38 g/cm3. 70 Table 4.7 Parameters used to study in-target decay. The production rates are calculated using the Liège cascade with 109 incident protons at 480 MeV and scaled to 1 mA. 73 viii List of Figures Figure 1.1 The Chart of Nuclides, a nuclear analogue to the periodic table, depicts all known nuclei in terms of their proton and neutron number. The colour contours represent the half-lives of each isotope. Data from [12]. The valley of stability, in the center of chart, clearly deviates from the Z = N trend. 4 Figure 1.2 Decays along the chart of nuclides. Adapted from Nucleonica [13]. 4 Figure 1.3 Illustration of proton induced fission process. 6 Figure 1.4 Illustration of a proton induced spallation reaction. 7 Figure 1.5 Illustration of the fragmentation process. The nucleus absorbs the projectile which leads to an unstable configuration. The nucleus splits into two fragments, which may then undergo nucleon evapora- tion. 7 Figure 1.6 Approximate mass distribution of products due to the proton in- duced reactions from protons of differing energies impinging on 209Bi. Image from [15]. 8 Figure 1.7 The TRIUMF Isotope Separation and ACceleration (ISAC) facility [3]. 15 Figure 1.8 Cyclotron motion and production of high energy protons at TRIUMF. 16 Figure 1.9 Uranium carbide before and after processing for a uranium ISAC target. Reprinted from [27], with permission from Elsevier. 17 Figure 1.10 ISAC target containers. Reprinted from [29], with permission from Elsevier . 18 Figure 1.11 The ISAC Yield Station. Reprinted from [31], with permission of AIP publishing. 19 Figure 1.12 Yield measurement of 30Mg. Reprinted from [31], with permission from AIP Publishing. 20 Figure 1.13 Yields measured at the ISAC Yield Station, for all targets used dur- ing ISAC’s operating history. Data from [30]. 21 Figure 1.14 The GEANT4 framework [32]. 23 ix Figure 1.15 A simple intranuclear cascade scheme shows that a proton enters the nucleus and interacts with a nucleon. This interaction may cause scattering or ejection of the participants or generation of other par- ticles, such as a p-meson.
Details
-
File Typepdf
-
Upload Time-
-
Content LanguagesEnglish
-
Upload UserAnonymous/Not logged-in
-
File Pages110 Page
-
File Size-