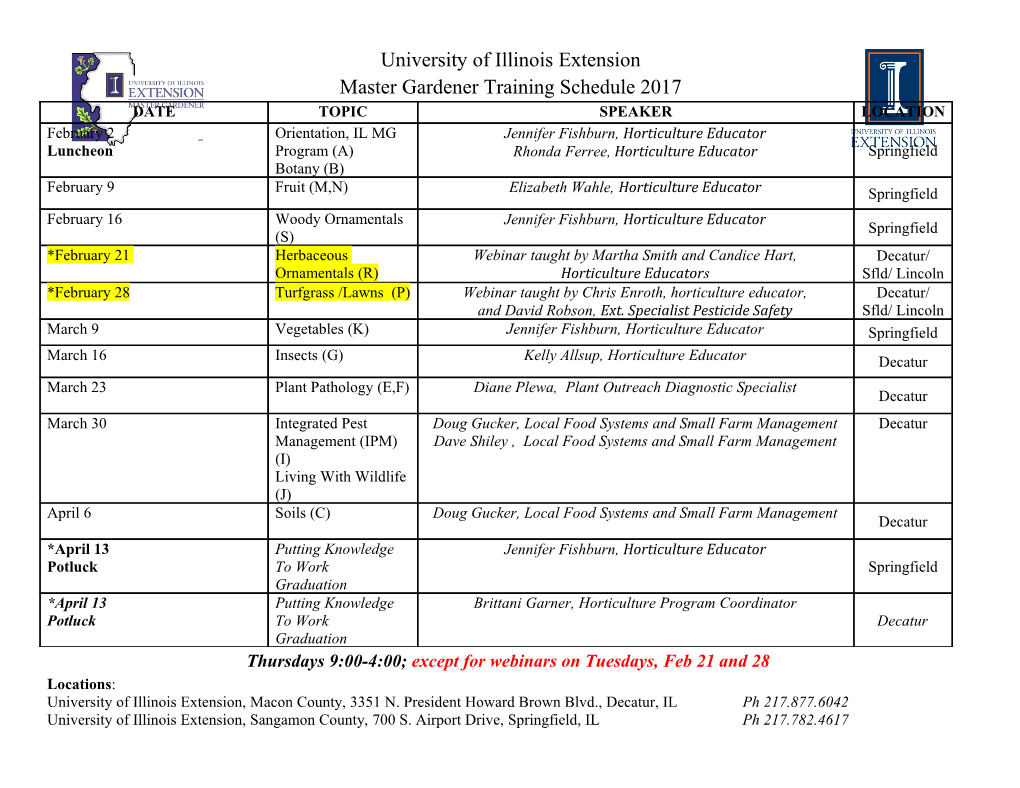
The Implied Volatility of Forward Starting Options: ATM Short-Time Level, Skew and Curvature Elisa Alòs Antoine Jacquier Jorge A. León May 2017 Barcelona GSE Working Paper Series Working Paper nº 988 The implied volatility of forward starting options: ATM short-time level, skew and curvature Elisa Al`os∗ Antoine Jacquier Dpt. d'Economia i Empresa Department of Mathematics Universitat Pompeu Fabra Imperial College London and Barcelona GSE London SW7 2AZ, UK c/Ramon Trias Fargas, 25-27 08005 Barcelona, Spain Jorge A. Le´ony Control Autom´atico CINVESTAV-IPN Apartado Postal 14-740 07000 M´exico,D.F., Mexico Abstract For stochastic volatility models, we study the short-time behaviour of the at-the-money implied volatility level, skew and curvature for forward- starting options. Our analysis is based on Malliavin Calculus techniques. Keywords: Forward starting options, implied volatility, Malliavin cal- culus, stochastic volatility models JEL code: C02 1 Introduction Consider two moment times s > t. A forward-start call option with maturity T > s allows the holder to receive, at time s and with no additional cost, a call option expirying at T , with strike set equal to KSs, for some K > 0. So, the option life starts at s, but the holder pays at time t the price of the option. Some classical applications of forward starting options include employee stock options and cliquet options, among others (see for example Rubinstein (1991)). Under the Black-Scholes formula, a conditional expectation argument leads to show that the price of a forward starting option is the price of a plain vanilla ∗Supported by grants ECO2014-59885-P and MTM2016-76420-P (MINECO/FEDER, UE). yPartially supported by the CONACyT grant 220303 1 option with time to maturity T −t. In the stochastic volatility case, a change-of- measure links the price of the forward option with the price of a classical vanilla (see, for example, Rubinstein (1991), Musiela and Rutkowski (1997), Wilmott (1998), or Zhang (1998)). For stochastic volatility models, change of numeraire techniques can be applied to obtain a closed-form pricing formula in the context of the Heston model (see Kruse and N¨ogel(2005)). The implied volatility surface for forward starting options exhibits substan- tial differences to the classical vanilla case (see for example Jacquier and Roome (2015)). This paper is devoted to the study of the at-the-money (ATM) short time limit of the implied volatility for forward starting options. More precisely, we will use Malliavin Calculus techniques to compute the ATM short-time limit of the implied volatility level, skew and curvature. In particular, we will see that -contrary to the classical vanilla case- the ATM short-time level depends on the correlation parameter (see Lemma 6 and Theorem 7). We will also prove that the skew depends of the Malliavin derivative of the volatility process in a similar way as for vanilla options, while the curvature (see Theorems 14 and 15) is of order O(T − s). The paper is organized as follows. Section 2 is devoted to introduce forward starting options and the main notation used troghout the paper. In Section 3 we obtain a decomposition of the option price that will allow us to compute, in Sections 4, 5 and 6, the limits for the ATM implied volatility level, skew and curvature. 2 Forward start option We will consider the Heston model for stock price on a time interval [0;T ] under a risk neutral probability P ∗ : ∗ p 2 ∗ dSt =rS ^ tdt + σtSt ρdWt + 1 − ρ Bt ; t 2 [0;T ]; (1) wherer ^ is the instantaneous interest rate (supposed to be constant), W ∗ and B∗ are independent standard Brownian motions defined on a probability space (Ω; F;P ∗) and σ is a positive and square-integrable process adapted to the ∗ ∗ filtration generated by W ∗. In the following we will denote by F W and F B the filtrations generated by W ∗ and B∗, respectively. Moreover we define F := ∗ ∗ F W _F B : It will be convenient in the following sections to make the change of variable Xt = log (St) ; t 2 [0;T ]: Now, we consider a point s 2 [0;T ] : We want to evaluate the following option price: −r^(T −t) ∗ XT α Xs Vt = e Et e − e e + ; (2) ∗ where E denotes the conditional expectation given Ft and α is a real constant. Notice that if t ≥ s this is the payoff of a call option, while in the case t < s this defines a forward start option. We will make use of the following notation 2 • BS(t; x; K; σ) will represent the price of a European call option under the classical Black-Scholes model with constant volatility σ, current log stock price x, time to maturity T − t; strike price K and interest rater: ^ Remember that in this case x −r^(T −t) BS(t; x; K; σ) = e N(d+) − e KN(d−); where N is the cumulative probability function of the standard normal law and x − ln K +r ^(T − t) σ p d± := p ± T − t: σ T − t 2 •L BS (σ) will denote the Black-Scholes differential operator (in the log vari- able) with volatility σ : @ 1 @2 1 @ L (σ) = + σ2 + r^ − σ2 − r:^ BS @t 2 @x2 2 @x It is well known that LBS (σ) BS (·; ·; K; σ) = 0: 2 • G(t; x; K; σ) := (@xx − @x)BS(t; x; K; σ): 2 • H(t; x; K; σ) := @x(@xx − @x)BS(t; x; K; σ): • BS−1(a) := BS−1 (t; x; K; a) denotes the inverse of BS as a function of the volatility parameter. • α∗ :=r ^(T − s) We recall the following result, that can be proved following the same argu- ments as in that of Lemma 4.1 in Al`os,Le´onand Vives (2007) W Lemma 1 Let 0 ≤ t ≤ s; u < T and Gt := Ft _FT : Then for every n ≥ 0; there exists C = C(n; ρ) such that a) If u ≤ s − 1 (n+1) Z T ! 2 n Xu 2 jE (@x G (u; Xu;Mu; vu)j Gt)j ≤ CE e Gt σs ds : s b) If u ≥ s − 1 (n+1) Z T ! 2 n Xs 2 jE (@x G (u; Xu;Mu; vu)j Gt)j ≤ CE e Gt σs ds : s 3 3 A decomposition formula for forward option prices We will stand for ∗ α Xs • Mt := Et e e , t 2 [0;T ]. We observe that Z t α rs^ X0 α r^(s−u) Xu ∗ p 2 ∗ Mt = e e e + σu1[0;s](u)e e e ρdWu + 1 − ρ dBu 0 Z t^s α r^(s−u) Xu ∗ p 2 ∗ = M0 + e σue e ρdWu + 1 − ρ dBu : 0 1 2 Yt • vt := T −t ; with Z T Z T 2 2 Yt := σu1[s;T ](u)du = σudu t t_s p p Notice that, if t < s, vt T − t = vs T − s: We will need the following hypotheses: (H1) There exist two constants 0 < c < C such that c < σt < C, for all t2 [0;T ], with probability one. (H2) σ; σeX 2 L2;2 \ L1;4: Now we are in a position to prove the following theorem, that allows us to identify the impact of correlation in the forward option price Theorem 2 Consider the model (1) and assume that hypotheses (H1) and (H2) hold. Then, for all 0 ≤ t ≤ s ≤ T , ∗ α Vt = Et exp(Xt)BS (s; 0; e ; vs) Z T ρ −r^(u−t) W ∗ + e H(u; Xu;Mu; vu)σuΛu du 2 s s ρ Z ∗ α −r^(u−t) Xu W + G(s; 0; e ; vs) e e σuΛu du : 2 t W ∗ R T W ∗ 2 where Λu := u_s Du σθ dθ: ∗ α Xs Proof. Remember that Mt := Et e e and notice that −r^(T −t) ∗ XT α Xs −r^(T −t) ∗ XT Vt = e Et e − e e + = e Et e − MT + −r^(T −t) = e BS(T;XT ;MT ; νT ): 4 Therefore, the anticipating It^o'sformula for the Skorohod integral (see for ex- ample Nualart (2006)) allows us to write ∗ −rT^ Et e BS(T;XT ;MT ; vT ) " Z T ∗ −rt^ −ru^ = Et e BS(t; Xt;Mt; vt) − r^ e BS(u; Xu;Mu; vu)du t Z T −ru^ @BS + e (u; Xu;Mu; vu)du t @u Z T 2 −ru^ @BS σu + e (u; Xu;Mu; vu) r^ − du t @x 2 Z T 2 1 −ru^ @ BS 2 + e 2 (u; Xu;Mu; vu)σudu 2 t @x Z T 2 −ru^ @ BS + e (u; Xu;Mu; vu)d hX; Miu t @x@K Z T 2 1 −ru^ @ BS + e 2 (u; Xu;Mu; vu)d hM; Miu 2 t @K Z T 2 1 −ru^ @ @ 2 2 + e 2 − BS(u; Xu;Mu; vu) vu − σu1]s;T ](u) du 2 t @x @x Z T 2 1 −ru^ @ @ @ W ∗ + e 2 − BS(u; Xu;Mu; vu)σuρΛu du 2 t @x @x @x T 2 # 1 Z @ @ @ ∗ −ru^ α r^(s−u) Xu W + e 2 − BS(u; Xu;Mu; vu)σue e e ρΛu 1[0;s](u)du : 2 t @K @x @x 5 That is, using t ≤ s, ∗ Vt = Et BS(t; Xt;Mt; vt) Z T −r^(u−t) + e LBS (vu) BS(u; Xu;Mu; vu)du t Z T 2 1 −r^(u−t) @ @ 2 2 + e 2 − BS(u; Xu;Mu; vu) σu − vu du 2 t @x @x Z T 2 −r^(u−t) @ BS + e (u; Xu;Mu; vu)d hX; Miu t @x@K Z T 2 1 −r^(u−t) @ BS + e 2 (u; Xu;Mu; vu)d hM; Miu 2 t @K Z T 2 1 −r^(u−t) @ @ 2 2 + e 2 − BS(u; Xu;Mu; vu) vu − σu1]s;T ](u) du 2 t @x @x Z T 2 1 −r^(u−t) @ @ @ W ∗ + e 2 − BS(u; Xu;Mu; vu)σuρΛu du 2 t @x @x @x s 2 1 Z @ @ @ ∗ −r^(u−t) α r^(s−u) Xu W + e 2 − BS(u; Xu;Mu; vu)σue e e ρΛu du : 2 t @K @x @x Thus, we get, for t ≤ s, ∗ Vt = Et BS(t; Xt;Mt; vt) Z T −r^(u−t) + e LBS (vu) BS(u; Xu;Mu; vu)du t Z T 2 1 −r^(u−t) @ @ 2 2 + e 2 − BS(u; Xu;Mu; vu) σu − σu1]s;T ](u) du 2 t @x @x Z T 2 −r^(u−t) @ BS + e (u; Xu;Mu; vu)d hX; Miu t @x@K Z T 2 1 −r^(u−t) @ BS + e 2 (u; Xu;Mu; vu)d hM; Miu 2 t @K Z T 2 1 −r^(u−t) @ @ @ W ∗ + e 2 − BS(u; Xu;Mu; vu)σuρΛu du 2 t @x @x @x s 2 1 Z @ @ @ ∗ −r^(u−t) α r^(s−u) Xu W + e 2 − BS(u; Xu;Mu; vu)σue e e ρΛu du : 2 t @K @x @x Now, taking into account the facts that LBS (vu)(BS)(u; Xu;Mu; vu) = 0; 2 α r^(s−u) Xu d hM; Xiu = σue e e 1[0;s](u)du; 6 2 2α 2^r(s−u) 2Xu d hM; Miu = σue e e 1[0;s](u)du; @2BS 1 @2BS @BS (t; x; K; σ) = − − (t; x; K; σ) @x@K K @x2 @x and @2BS 1 @2BS @BS (t; x; K; σ) = − (t; x; K; σ) ; @K2 K2 @x2 @x it follows that ∗ Vt = Et BS(t; Xt;Mt; vt) Z T 2 1 −r^(u−t) @ @ @ W ∗ + e 2 − BS(u; Xu;Mu; vu)σuρΛu du 2 t @x @x @x s 2 1 Z @ @ @ ∗ −r^(u−t) α r^(s−u) Xu W + e 2 − BS(u; Xu;Mu; vu)σue e e ρΛu du 2 t @K @x @x ∗ = Et BS(t; Xt;Mt; vt) Z T 2 1 −r^(u−t) @ @ @ W ∗ + e 2 − BS(u; Xu;Mu; vu)σuρΛu du 2 s @x @x @x Z s 2 1 −r^(u−t) @ @ @ W ∗ + e 2 − BS(u; Xu;Mu; vu)σuρΛu du 2 t @x @x @x Z s 2 1 −r^(u−t) @ @ @ W ∗ + e 2 − BS(u; Xu;Mu; vu)σuMuρΛu du : 2 t @K @x @x Since we have @ @2BS @BS exN 0(d ) d − (t; x; k; σ) = p + 1 − p + @x @x2 @x σ T − t σ T − t and @ @2BS @BS exN 0(d ) d − (t; x; k; σ) = p + p + ; @K @x2 @x Kσ T − t σ T − t then it is easy to see that ∗ Vt = Et BS(t; Xt;Mt; vt) Z T ρ −r^(u−t) W ∗ + e H(u; Xu;Mu; vu)σuΛu du 2 s Z s ρ −r^(u−t) W ∗ + e G(u; Xu;Mu; vu)σuΛu du : 2 t 7 Hence, due to BS(t; Xt;Mt; vt) p −α +r ^(T − s) vs T − s = exp(Xt)N p + vs T − s 2 p α −r^(T −s) −α +r ^(T − s) vs T − s −e exp(Xt)e N p − vs T − s 2 α = exp(Xt)BS (s; 0; e ; vs) and, for all u < s, p −α+^r(T −s) eXu N 0 p + vs T −s vs T −s 2 G(u; Xu;Mu; vu) = p vs T − s Xu α = e G(s; 0; e ; vs); the proof is complete.
Details
-
File Typepdf
-
Upload Time-
-
Content LanguagesEnglish
-
Upload UserAnonymous/Not logged-in
-
File Pages21 Page
-
File Size-