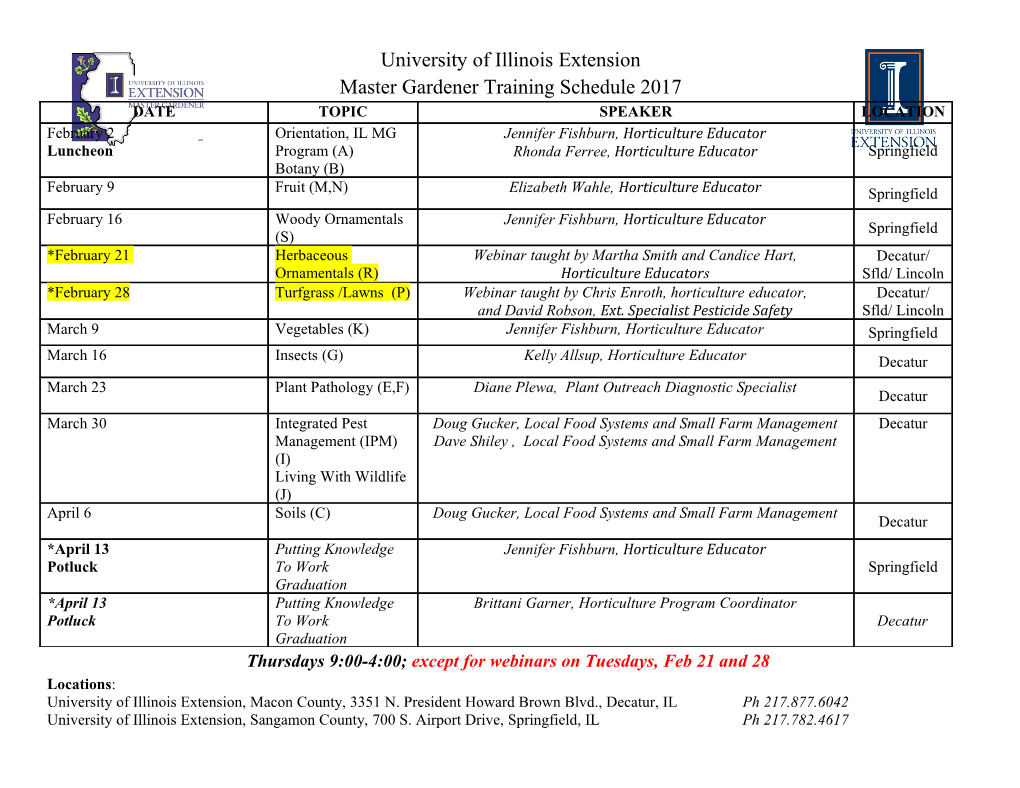
Member Recognition Issue VOL. 31, NO. 7 | J U LY 2 021 ’Taters versus Sliders: Evidence for a Long-Lived History of Strike-Slip Displacement along the Canadian Arctic Transform System (CATS) ’Taters versus Sliders: Evidence for a Long- Lived History of Strike-Slip Displacement along the Canadian Arctic Transform System (CATS) William C. McClelland, Dept. of Earth & Environmental Sciences, University of Iowa, Iowa City, Iowa 52242, USA; Justin V. Strauss, Dept. of Earth Sciences, Dartmouth College, Hanover, New Hampshire 03755, USA; Maurice Colpron, Yukon Geological Survey, Whitehorse, Yukon Y1A 2C6, Canada; Jane A. Gilotti, Dept. of Earth & Environmental Sciences, University of Iowa, Iowa City, Iowa 52242, USA; Karol Faehnrich, Dept. of Earth Sciences, Dartmouth College, Hanover, New Hampshire 03755, USA; Shawn J. Malone, Dept. of Geoscience, University of Wisconsin, Green Bay, Wisconsin 54311, USA; George E. Gehrels, Dept. of Geosciences, University of Arizona, Tucson, Arizona 85721, USA; Francis A. Macdonald, Earth Science Dept., University of California, Santa Barbara, California 93106, USA; John S. Oldow, Borealis, 200 E. Troxell Road, Oak Harbor, Washington 98277, USA, and Dept. of Geology, Western Washington University, Bellingham, Washington 98225, USA ABSTRACT the northwestern Alaskan and Canadian followed mafic magmatism associated with Recent field-based studies indicate that the Arctic margins provide the clearest rationale the Franklin Large Igneous Province at 720 northern margin of North America is best for the rotation model (Embry, 1990), which Ma (Macdonald et al., 2010; Cox et al., interpreted as a tectonic boundary that experi- is by far the most commonly expressed 2015). The basin is flanked to the north by enced a long, complex history of strike-slip mechanism (e.g., Hutchinson et al., 2017; Ordovician to Silurian clastic and subduction- displacement. Structures juxtaposing the Miller et al., 2018). In contrast, we explore related mafic and ultramafic rocks and alloch- Pearya and Arctic Alaska terranes with North the implications of a growing set of onshore thonous units of the Pearya terrane (Fig. 1; America are linked and define the Canadian observations that indicate that the northern Trettin, 1998). The Pearya terrane is domi- Arctic transform system (CATS) that accom- Laurentian margin has experienced a pro- nated by two assemblages juxtaposed in modated Paleozoic terrane translation, trun- tracted history of translation. This view is the Ordovician: a displaced peri-Laurentian cation of the Caledonian orogen, and shorten- bolstered by a variety of data that support crustal fragment that records early Neo- ing within the transpressional Ellesmerian models of Paleozoic large-magnitude terrane proterozoic (Tonian) and Ordovician conver- orogen. The structure was reactivated during translation through the Arctic region (Colpron gent margin magmatism (Malone et al., 2017, Mesozoic translational opening of the Canada and Nelson, 2009). Despite early calls for 2019) and a latest Neoproterozoic (Ediacaran) Basin. Land-based evidence supporting trans- large-magnitude sinistral offsets (e.g., Boreal to Ordovician mafic arc complex built on lation along the Canadian Arctic margin is fault of Bally in Kerr et al., 1982; Canadian Tonian basement (Majka et al., 2021). Steeply consistent with transform structures defined transcurrent fault of Hubbard et al., 1987; dipping faults juxtaposed Pearya with the by marine geophysical data, thereby provid- Porcupine fault of Oldow et al., 1989), the Laurentian passive margin by the Devonian ing a robust alternative to the current consen- Canadian Arctic margin generally has not (Trettin, 1998; Malone et al., 2019). Sub- sus model for rotational opening of the been viewed as a viable candidate for trans- sequently, units of both the Pearya terrane Canada Basin. form boundaries to accommodate evolution and Franklinian basin were deformed within of the Arctic region (e.g., Doré et al., 2016). the Devonian–Carboniferous Ellesmerian INTRODUCTION Results of our recent field studies on the fold belt and overlain by Carboniferous and Recent ocean- and land-based studies of northern margin of Laurentia challenge this younger deposits of the Sverdrup basin. the circum-Arctic region bring significant conclusion and support translation. Structures of the Ellesmerian fold belt extend advances in high-quality data to formulate westward to Prince Patrick Island where they, new models for the tectonic evolution of the CURRENT SETTING and Carboniferous to Mesozoic structures of Arctic margin (e.g., Pease and Coakley, 2018; The Canadian Arctic Islands expose a the Sverdrup basin, are truncated at a high Piskarev et al., 2019; Piepjohn et al., 2019). south to north transition from shallow marine angle by the present-day Arctic margin (Fig. Nevertheless, evolution of the Canada Basin deposits of the Paleozoic Arctic platform into 1; Harrison and Brent, 2005). remains one of the most enigmatic and con- deep-water rocks of the late Proterozoic to Autochthonous strata of the Laurentian tentious topics of the Arctic. Two end-mem- early Paleozoic Franklinian basin that were margin in northern Yukon are juxtaposed ber models for the Mesozoic opening of the deformed in the Devonian and overlain by late with peri-Laurentian platform and basinal Canada Basin invoke Arctic Alaska (1) rift- Paleozoic and early Mesozoic rocks of the strata of the North Slope subterrane of Arctic ing and rotating (’taters) from or (2) translat- Sverdrup basin. Rocks of the Franklinian Alaska (Macdonald et al., 2009; Strauss et al., ing (sliders) along the Canadian Arctic basin were deposited after the Neoproterozoic 2019a, 2019b; Colpron et al., 2019) on a near- margin (Fig. 1 inset). Late Paleozoic and breakup of Rodinia and rifting along the vertical fault zone broadly referred to as the Mesozoic stratigraphic correlations between northern Laurentian margin, which closely Porcupine shear zone (Fig. 1; von Gosen et al., GSA Today, v. 31, https://doi.org/10.1130/GSATG500A.1. CC-BY-NC. 4 GSA Today | July 2021 150°E150° E 180° E E Seward ° °EE N °NN 6060°E Peninsula 0°N E 90°90 E 60°N 8080°N 70°70°N7 120° 120° Podvodnikov Basin s Southwestern Brook Lomonoso Paleogene Gak Aleutia subterranes Chukchi Borderland fault FarewelFarewelll Amerasian Basin kel n Makarov Paleozoic v Denali Kaltag fault R Rang Basin id Rid ge 150°W Canada 30°E T ge rench e Eurasian Basin area of inferred oceanic crust 30°W Svalbard Porcupin Alpha NorthNorth SlopSlopeSlopee Amerasia e Basin Ridge subterranesubterran e Harder Fjord Alaska Yukon e South ansform System de Geer Pacific Ridg Canadian Arctic Transform System St Elias Tuk nana north a Pearya Atlantic Ocean T Prince Patrick Island Banks Caledo r Yukon-Tanana north Island Sverdrup T Prince of BC basin Franklinian Ocean 0° NW Wales a Basin Charlotte nana south Devonian n ian Germania Land clastic wedge e AlexanderAlexande Tintin deformation zone kon-Tanana south 1 2 deformationdefo Queen Yu land EllesmerIslandIs Mtns Nunavut Mackenzie r W Greenland mati 0° Storstrømmen BankBankss 9 Island on shear zone n ont frontfronfr t 120°120°WW n Arctic Alaska terraneBaffin 0500 pole of Bay fronfr W o CordilleranCordillera Insular-Arctic terranes n rotation N t 60°W km 60°W 60° deformationdeformatio 70°70°NN Figure 1. Generalized terrane map showing the location of the Canadian Arctic transform system, geophysically defined features in the Canada Basin, and terrane distribution on the Arctic and Cordilleran margins of Laurentia (modified after Colpron et al., 2019). Insets show simplified (1) rotation and (2) trans- lation models (from Patrick and McClelland, 1995). 2019). The North Slope subterrane was incor- Structures that accommodated translation the Porcupine shear zone while it was an porated into the greater Arctic Alaska terrane of Pearya project eastward to faults with active lithosphere-scale transform (Ward et and juxtaposed with the Laurentian margin similar timing and kinematics in Svalbard al., 2019). Strike-slip basin sedimentation by the Carboniferous (Strauss et al., 2013). (Fig. 1; Mazur et al., 2009). Although com- may be recorded by the newly recognized South-directed Late Devonian–Carboniferous monly linked with strike-slip faults in the Devonian–Carboniferous Darcy Creek for- structures on the north side of this boundary Caledonides (e.g., Storstrømmen shear zone; mation within the Porcupine shear zone mark the probable offset western continuation Fig. 1), we suggest that faults in Svalbard (Faehnrich et al., 2021). of the Ellesmerian orogen (Oldow et al., 1987). truncate the Caledonian structures and con- Linking strike-slip structures across tinue eastward to Scandinavia as the de Geer Svalbard and northern Ellesmere Island to PALEOZOIC TERRANE ACCRETION transform (Fig. 1; Lundin and Doré, 2019). Yukon and Alaska on the basis of orienta- AND TRANSLATION ON THE PEARYA The Harder Fjord fault zone, a long-lived tion, timing, and kinematics defines a AND PORCUPINE SHEAR ZONES steep structure that juxtaposes Ediacaran arc throughgoing Paleozoic fault system on the Models that invoke terrane translation from rocks with the Franklinian margin on North northern Laurentian margin (Fig. 1), referred the Arctic domain to the Cordilleran margin Greenland (Rosa et al., 2016), is similar to to here as the Canadian Arctic margin (e.g., Northwest Passage model; Colpron and faults in Pearya and Svalbard (Fig. 1). transform system (CATS). Recognition of Nelson, 2009) require a transcurrent bound-
Details
-
File Typepdf
-
Upload Time-
-
Content LanguagesEnglish
-
Upload UserAnonymous/Not logged-in
-
File Pages9 Page
-
File Size-