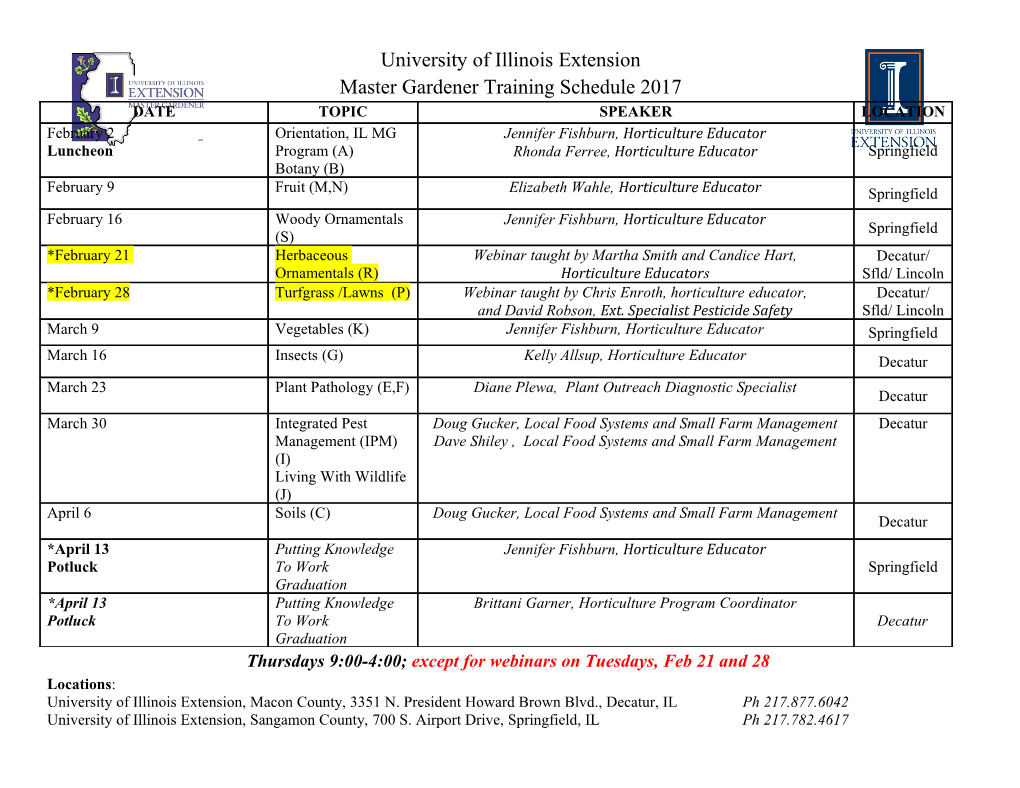
Reviews in Mineralogy & Geochemistry Vol. 80 pp. 61-164, 2015 4 Copyright © Mineralogical Society of America Characterization and Analysis of Porosity and Pore Structures Lawrence M. Anovitz Chemical Sciences Division Oak Ridge National Laboratory Oak Ridge, Tennessee, USA [email protected] David R. Cole School of Earth Sciences The Ohio State University Columbus, Ohio, USA [email protected] INTRODUCTION Porosity plays a clearly important role in geology. It controls fl uid storage in aquifers, oil and gas fi elds and geothermal systems, and the extent and connectivity of the pore structure control fl uid fl ow and transport through geological formations, as well as the relationship between the properties of individual minerals and the bulk properties of the rock. In order to quantify the relationships between porosity, storage, transport and rock properties, however, the pore structure must be measured and quantitatively described. The overall importance of porosity, at least with respect to the use of rocks as building stone was recognized by TS Hunt in his “Chemical and Geological Essays” (1875, reviewed by JD Dana 1875) who noted: “Other things being equal, it may properly be said that the value of a stone for building purposes is inversely as its porosity or absorbing power.” In a Geological Survey report prepared for the U.S. Atomic Energy Commission, Manger (1963) summarized porosity and bulk density measurements for sedimentary rocks. He tabulated more than 900 items of porosity and bulk density data for sedimentary rocks with up to 2,109 porosity determinations per item. Amongst these he summarized several early studies, including those of Schwarz (1870–1871), Cook (1878), Wheeler (1896), Buckley (1898), Gary (1898), Moore (1904), Fuller (1906), Sorby (1908), Hirschwald (1912), Grubenmann et al. (1915), and Kessler (1919), many of which were concerned with rocks and clays of commercial utility. There have, of course, been many more such determinations since that time. There are a large number of methods for quantifying porosity, and an increasingly complex idea of what it means to do so. Manger (1963) listed the techniques by which the porosity determinations he summarized were made. He separated these into seven methods for obtain total porosity, sixteen for determining apparent (connected) porosity and fi ve where the methods used were, for one reason or another, uncertain. Most of the total porosity measurements are variations on bulk volume/grain volume or bulk density/grain density approaches, and the apparent porosity measurements were made by variations of absorption methods for different fl uids or gases. These techniques were augmented just before and 1529-6466/15/0080-0004$15.00 http://dx.doi.org/10.2138/rmg.2015.80.04 62 Anovitz & Cole soon afterward Manger’s report by point counting approaches (Chayes 1956; Manger 1963; Gazzi 1966; Dickinson 1970; Folk 1974). For downhole petrophysical analysis Archie’s Law (Archie 1942, 1947, 1950, 1952; cf. Rider 1999; Ellis and Singer 2008; Peters 2012; Tiab and Donaldson 2012) provided a relationship between electrical conductivity/resistivity porosity and brine saturation, and porosity information is also provided by density, sonic and neutron logs (Peters 2012; Tiab and Donaldson 2012). Porosity, itself, is a rather easy parameter to defi ne—the fraction of void volume over total volume - but certainly not so easy to quantify. The reason is that “void” space expressed in earth materials can span over 8 orders of magnitude in length scale—i.e., nanometer to 10s or even 100s cm or larger. As we describe below there is really no one method that can adequately cover this enormous range in scale. Whether rocks are formed by crystallization of a melt, or deposition of sediments in a stream, lake or ocean, they initially contain some inherent primary porosity. This porosity can then be modifi ed by a variety of processes such as deformation (including fracture), metamorphism, hydrothermal alteration, diagenesis and weathering, producing secondary or fracture porosity. In a hydrologic sense we have two types of porosity—effective or open porosity that permits fl ow of fl uids or volatiles and ineffective or closed porosity that does not. As our ability to quantify the nature of porosity in complex heterogeneous matrices has improved through the application of sophisticated techniques such as electron microscopy and X-ray and neutron scattering, so too has our need for a fi ner breakdown in the defi nition of pore types. Leaders in the fi eld such as Loucks (Loucks et al. 2012) have expanded our understanding of porosity by necessity as we examine in detail very fi ne-grained, tight formations such as shale for hydrocarbon potential. In this context we now have a hierarchy of pores types that range from interparticle and intraparticle mineral-matrix pores, to organic matter pores to fracture pores. The primary goal in pore assessment is to quantify these pores, not just in terms of shape and size, but how they contribute to the overall fabric of the rock and its ability to transmit fl uids, and the bulk physical properties of the rock itself. The goal of this paper is to summarize, in a manner the reader can use for future experimental work, many of the techniques for analyzing the porosity of rocks or other porous materials. The paper is divided into four sections. The fi rst summarizes petrophysical approaches. While some of these have been known since the early work just mentioned, such as direct measurements of volume and density, pore saturation, gas expansion and mercury intrusion, others, like focused ion beam milling, QEMSCAN imaging, and nuclear magnetic resonance approaches are relatively new and may be less familiar. The next section of this paper discusses scattering methods for porosity analysis. While the fi rst scattering studies of rock materials were published in the 1980’s (e.g., Hall et al. 1983), it is only recently (cf. Anovitz et al. 2009) that these have been used to study porosity changes in larger geologic contexts. This section discusses the theoretical basis of scattering experiments, sample preparation, the nature of scattering instruments, contrast matching experiments, data reduction and analysis. Tomographic approaches are covered elsewhere in this volume (Noiriel 2015, this volume). The third section of the paper discusses image analysis approaches other than the optical petrophysical techniques discussed in the fi rst section. These include methods and limitations of obtaining binary pore/rock images, calculations to use imaging to extend the size range of scattering data using one- and two-point correlations, and the potential utility of three- point correlation analysis. Fractal approaches to image quantifi cation, including mono- and multifractal analysis, lacunarity and succolarity, as well as other types of correlations are also discussed. Characterization and Analysis of Porosity and Pore Structures 63 Finally, it should be kept in mind that different techniques are based on different inherent assumptions. This is true both in terms of how they function and what they are trying to, or are capable of measuring. Thus, different approaches to pore analysis may, in fact, be likely to yield differing results. This may advantageous, as the combination of several techniques, each with its own goals, may elucidate differing features of the pore structure, but few such comparisons, especially involving scattering studies, are available. In the concluding section of the paper, therefore, a few examples comparing the results of pore structure analysis using different techniques are considered. PETROPHYSICAL APPROACHES Conventional petrophysics involves the characterization of reservoirs for their hydrocarbon potential through the quantitative assessment of rock properties. Of primary interest is how pores (and not uncommonly fractures) are interconnected, thus controlling migration and accumulation of hydrocarbon fl uids and gases. The key properties of interest include lithology (e.g., mineralogy, grain fabric), water saturation, porosity, permeability and density. Three broad categories of measurements are typically made, (a) on core or crushed rock, (b) within the bore hole via well logging tools and (c) seismic. In this chapter we will briefl y address only those methods used in (a) and (b) that lead to quantifi cation of porosity or pore features. In category (a) we can further subdivide the methods into direct measurements on solids and indirect via imaging of a rock core or chip. A number of these overlap with respect to length scales but yet complement methods such as neutron scattering (Fig. 1) Figure 1. Methods used to determine porosity and pore size distribution (PSD). Direct methods Several methods can be employed to directly measure rock porosity: (1) saturation or imbibition, (2) buoyancy, (3) gas expansion (He porosimetry), (4) gas adsorption (BET) and (5) mercury intrusion porosimetry (e.g., Tiab and Donaldson 2004; Sondergeld et al. 2010; Clarkson et al. 2012c). All fi ve of these methods only measure the effective (connected) porosity of the rock sample, similar in a general way to the contrast-match method used in neutron scattering to assess connected pores (see section on Contrast Matching below). 64 Anovitz & Cole Saturation (or imbibition). In the saturation (or imbibition) method, a clean, dry rock is weighed prior to full saturation with a wetting fl uid (Wdry). Typically toluene or dichloromethane have been used in the past but more recently it has been common to saturate a rock with a synthetic brine that has a cation and anion composition similar to that observed in the reservoir of interest. The weight of the saturated sample (Wsat) is determined after excess brine is removed from the surface of the sample. The bulk volume of the sample (Vbulk) in the form of a cylinder or cube can be determined by geometric means using a caliper. One must also know the density of the saturating fl uid (fl uid) or determine it by weighing a known volume of the fl uid.
Details
-
File Typepdf
-
Upload Time-
-
Content LanguagesEnglish
-
Upload UserAnonymous/Not logged-in
-
File Pages104 Page
-
File Size-