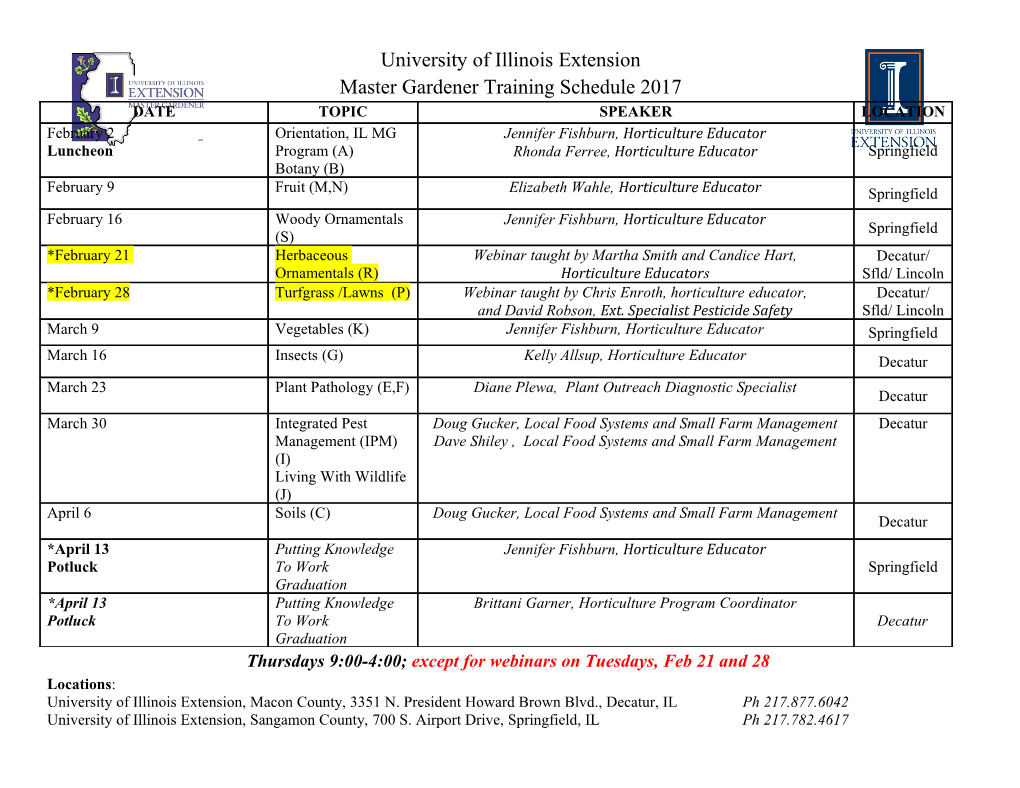
XMM-Newton 2019 Science Workshop “Astrophysics of hot plasma in extended X-ray sources” 2019-06-12 European Space Astronomy Centre (ESAC), Madrid, Spain Modelling and simulations of supernova remnants Gilles Ferrand Research Scientist Astrophysical Big Bang Laboratory (ABBL) and Interdisciplinary Theoretical and Mathematical Sciences Program (iTHEMS) + A. Decourchelle, S. Safi-Harb + S. Nagataki, D. Warren, M. Ono, F. Röpke, I. Seitenzhal Modelling and simulations of supernova remnants with a focus on morphological studies Introduction to SNRs Structure and evolution of a remnant Multi-wavelength emission 1. SNRs as particle accelerators Hydro-kinetic coupling for diffusive shock acceleration (DSA) Non-equilibrium ionization and thermal emission from the plasma Magnetic field amplification and non-thermal emission from the particles 2. SNRs as probes of the explosion From the supernova to the remnant: Cas A, Tycho Example: the N100 supernova model X-ray image analysis Supernova remnants 0.1 SNRs as a key link between stars and the ISM Tycho’s SNR age: ∼440 yr distance: 1.5–5 kpc enrichment in heavy elements size: 8’ ∼3–12 pc average stars: up to C-O massive stars: up to Fe supernovae: above Fe hot, turbulent metal-rich plasma injection of energy heating of the gas hydrodynamic turbulence magnetic field amplification multi- wavelength composite image: - X-rays acceleration (Chandra) of particles - Optical large, powerful (Calar Alto) shock wave most favoured Galactic sources - infrared 15 (Spitzer) up to the knee (< 10 eV) 0.2 Classification of SNRs from radio + X-ray observations SNR 0509-67.5 W49B G21.5-0.9 Crab Nebula shell composites filled-centre “mixed morphology” plerionic isolated/shell-less = thermal composite: composite (= non- pulsar wind nebula centrally peaked thermal composite): = PWN (= plerion) PWN inside shell or (can be both) bow shock nebula 0.3 The evolution of a supernova remnant radius R non-radiative radiative momentum-driven values given for pressure-driven 1.4 solar masses 46 pc of ejecta with kinetic energy of 1051 erg, expanding in a Sedov-Taylor medium of density 7 pc 0.1 cm-3 ejecta-dominated 600 yr 30 000 yr time t G1.9+0.3 Tycho RCW 86 Simeis 147 Monoceros Loop 140 yr 440 yr 2,000–10,000 yr ∼40,000 yr ∼300,000 yr 0.4 The structure of a young shell SNR Tycho’s SNR 0.95 – 1.26 keV 1.63 – 2.26 keV as seen by Chandra 4.10 – 6.10 keV at age 433 yr Warren et al 2005 0.5 SNR broad-band emission A&A 516, A62 (2010) SN 1006 Fig. 6. Azimuthal profile obtained from HESS data and XMM-Newton data in the 2–4.5 keV energy band and smoothed to HESS PSF, re- stricted to radii 0.12◦ r 0.36◦ from the centre of the SNR. Azimuth ≤ ≤ 0◦ corresponds to East, 90◦ corresponds to North, 180◦ to West and 90◦ to South. − radio optical X Fig. 4. HESS γ-ray image ofgamma SN 1006. The linear colour scale is in units 2 2 of excess counts per π (0.05◦) .Pointswithin(0.05◦) are correlated. The white cross indicates× the geometrical centre of the SNR obtained from XMM data as explained in the text and the dashed circlesE cor- respond to R dR as derived from the fit. The white star shows the centre of the circle± encompassing the whole X-ray emission as derived keV by Rothenflug et al. (2004) andTeV the white triangle the centre derived by cm µm Cassam-Chenaï et al. (2008) from Hα data. The white contours corre- spond to a constant X-ray intensity as derived from the XMM-Newton λ flux map and smoothed to the HESS point spread function, enclosing respectively 80%, 60%, 40% and 20% of the X-ray emission. The inset shows the HESS PSF using an integration radius of 0.05◦. synchrotron Balmer lines atomic lines of Inverse Compton ? in B field forbidden lines heavy elements pion decay ? Fig. 7. Differential energy spectra of SN 1006 extracted from the two + synchrotron regions NE and SW as defined in Sect. 2.Theshadedbandscorrespond to the range of the power-law fit, taking into account statistical errors. Table 2. Fit results for power-law fits to the energy spectra. Region Photon index ΓΦ(>1TeV) 12 2 1 (10− cm− s− ) NE 2.35 0.14stat 0.2syst 0.233 0.043stat 0.047syst SW 2.29 ± 0.18 ± 0.2 0.155 ± 0.037 ± 0.031 GeV e- blast wave hot ejecta > TeV e- ? ± stat ± syst ± stat ± syst Fig. 5. Radial profile around the centre of the SNR obtained from HESS data and XMM-Newton data in the 2–4.5 keV energy band smoothed to + TeV e- HESS PSF. > TeV p ? The spectra for the NE and SW regions are compatible with Γ power law distributions, F(E) E− ,withcomparablephoton indices Γ and fluxes. Confidence∝ bands for power-law fits are centred on 143.6◦ 6.1◦ (SW region) and 29.3◦ 4.0◦ (NE − ± ± shown in Fig. 7 and Table 2.Theintegralfluxesabove1TeV region) and with similar widths of 33.8◦ 7.0◦and 27.9◦ 4.0◦. ± ± correspond to less than 1% of the Crab flux, making SN 1006 reviews (high energies perspective): Reynolds 2008, Vink 2012 one of the faintest known VHE sources (Table 2). The derived 4. Spectral analysis fluxes are well below the previously published HESS upper lim- its (Aharonian et al. 2005). The observed photon index Γ 2.3is Differential energy spectra of the VHE γ-ray emission were de- somewhat steeper than generally expected from diffusive≈ shock rived for both regions above the energy threshold of 260 GeV. acceleration theory and may indicate that the upper cut-off of the These regions correspond to 80% of the X-ray emission∼ (after high-energy particle distribution is being observed; however, the smearing with the HESS PSF) and therefore slightly underesti- uncertainties on the spectrum preclude definitive conclusions on mate the TeV emission of the full remnant. this point. Page 4 of 7 SNRs as particle accelerators 1.1 Efficient particle acceleration SNRs are widely believed to be the main producers of CRs in the Galaxy • Available energy budget — but can we reach the knee? • Known acceleration mechanism — but what spectrum? • Observed energetic electrons — and protons? If CRs are efficiently accelerated by the blast wave, it must impact its dynamics - fluid becomes more compressible - energy leaks from the system → non-linearly coupled system CRs are a key ingredient of SNRs 1.2 Diffusive shock acceleration: the coupled system IR Opt radio UV X γ X shock wave cosmic-rays injection, acceleration (thermal magnetized (non-thermal shock modification plasma) population) conservation laws damping particle distribution: heating ∂X 2 +div(F (X)) = 0 n (x, t)= f (x, p, t)4πp dp ∂t Zp ρ ρu transport equation: X = ρu F (X)= ρu u + pI 0 1 0 ⌦ 1 ∂f ∂ ∂ ∂f 1 ∂p3f ∂u P (e + P ) u generationdiffusion, (instabilities) injection, + (uf)= D + 2 stochastic acceleration∂t ∂x ∂x ∂x 3p ∂p ∂x @ A @ A ✓ ◆ magnetic waves hydrodynamic (collective movements kinetic treatment of charges) treatment reviews on DSA : Drury 1983, Jones and Ellison 1991, Malkov and Drury 2001 on numerical techniques for DSA: Marcowith et al (in prep) 1.3 Numerical simulations: hydro + kinetic slice of log(density) Teyssier 2002, Chevalier 1982, 1983 Fraschetti et al 2010 SNR initialization: SNR evolution: self-similar profiles 3D hydro code from Chevalier ramses parameters: Tycho (SN Ia) tSN = 440 years shock back-reaction: 51 ESN = 10 erg diagnostics varying gamma n =7,Mej =1.4M Ellison et al 3 Ferrand et al 2010 s =0,n =0.1 cm− H,ISM 2007 (A&A 509 L10) particle acceleration: non-linear model of Blasi Blasi et al 2002, 2004, 2005 Anne + Caprioli 2008, 2009 Decourchelle Head of Astrophysics Dpt. Using a comoving grid to at CEA Saclay / Irfu factor out the expansion 1.11.4 Computing the emission from the SNR Thermal emission from the shocked plasma Samar Safi-Harb Prof. at the University of Manitoba test-particle case Canadian Research Chair Ferrand, Decourchelle, Safi-Harb 2012 Non-thermal emission from the accelerated particles modified shock Ferrand, Decourchelle, Safi-Harb 2014 with magnetic field amplification 1.5 Hydro- and thermodynamics of the plasma Thermal emission in each cell depends on: • plasma density n2 • electron temperature T e n progressive equilibration with protons temperature Tp via Coulomb interactions • ionization states fi(Z) Tp computation of non-equilibrium ionization - solving the coupled time-dependent system of equations Patnaude et al 2009, 2010 - using the exponentiation method ⌧ in post-processing I t slices at t = 500 yr from a 10243 simulation with particle τI = n(t0).dt0 back-reaction ZtS Smith & Hughes 2010 all these parameters depend on the history of the material after it was shocked. Ferrand, Decourchelle, Safi-Harb 2012 1.6 Thermal emission Ferrand, Decourchelle, Safi-Harb 2012 using an emission code adapted from Mewe, with rates from emissivity [erg/s/cm3/eV] Arnaud 1024^3 cells t = 500 yr energy [eV] test particle vs. back-reaction test particle vs. back-reaction test particle vs. back-reaction 1.7 Magnetic field and radiative losses Non-thermal emission in each cell depends on: • pion decay: plasma density n(x, t) • synchrotron: magnetic field B ( x, t) n (amplified at the shock, then frozen in the flow) • Compton: ambient photon fields (CMB) B Note: the acceleration model gives the CR spectra just behind the shock fp(p, x, t) ,fe(p, x, t) they must be transported to account for losses: slices at t = 500 yr from a ✓ 10243 simulation without ⇥(x, t) particle back-reaction • adiabatic decompression α = and MF amplification ⇥(xS,tS) t 2 1 • radiative losses Θ B α 3 dt / ZtS Ferrand, Decourchelle, Safi-Harb 2014 1.8 Non-thermal emission Ferrand, Decourchelle, Safi-Harb 2014 synchrotron (e) pion decay (p) using the emission code from P.
Details
-
File Typepdf
-
Upload Time-
-
Content LanguagesEnglish
-
Upload UserAnonymous/Not logged-in
-
File Pages34 Page
-
File Size-