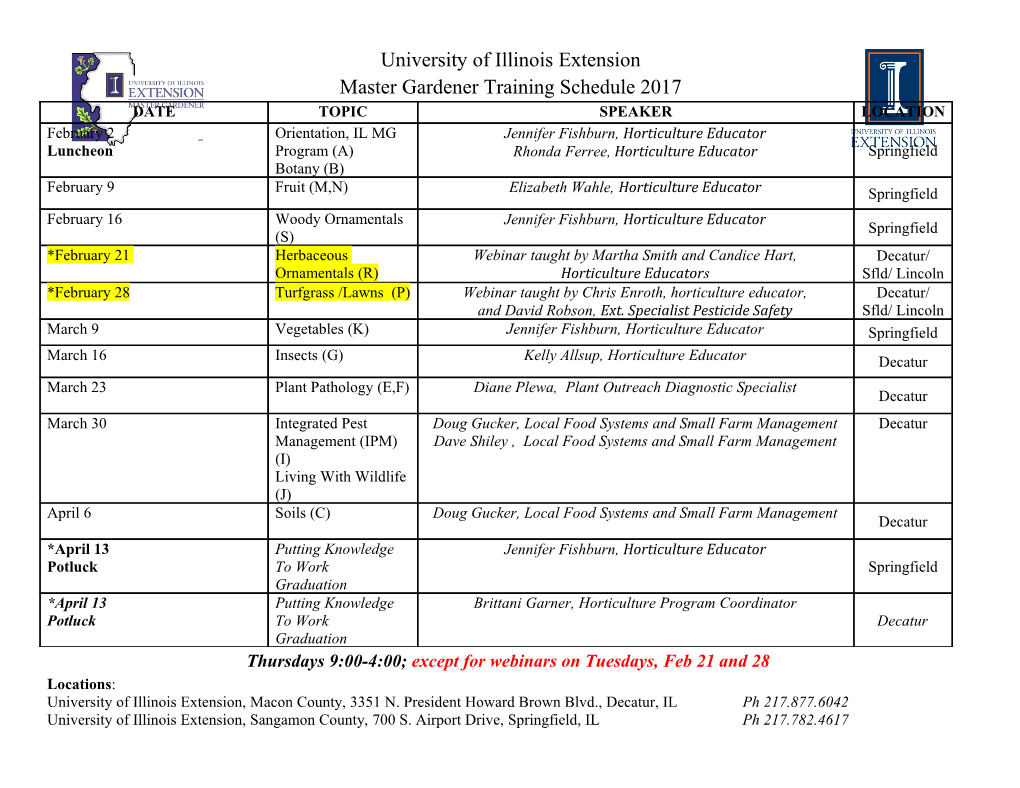
Ribozyme – Earlier we firmly believed that proteins were the only catalytic macromolecules in biology. However, this conception was overturned with the discovery of the first catalytic RNA molecules, or ribozymes. In the early 1980s, research groups led by Sidney Altman and Thomas Cech independently found that RNAs can also act as catalysts for chemical reactions. Just like enzymes, some RNA sequences form complex tertiary structures and play the role of biological catalysts. As we already discussed about self-splicing introns in which the intron RNA sequence catalyzes its own cleavage out of the RNA precursor without the need for a spliceosome. Such catalytic RNA molecules are called ribozymes (a name that is clearly fashioned on ‘enzymes’, i.e. protein catalysts). Like enzymes they have an active site, a binding site for a substrate and a binding site for a co-factor such as metal ion. In the majority of cases the substrates of ribozymes are RNA, and most ribozymes serve an RNA- processing function. They have been extensively studied in rRNA of a ciliate protozoan Tetrahymena thermophila and are also found in fungal mitochondria, in phage T4 and E. coli bacteria. The simplest are the nucleolytic ribozymes. There are four distinct classes of nucleolytic ribozymes that have unrelated structures: hammerhead and hairpin ribozymes, mostly found in plant viruses, the Varkud satellite (VS) ribozyme in fungal mitochondria and the hepatitis delta virus (HDV) ribozyme, which is present in a human pathogen. As we discussed already in earlier lectures, Group 1 introns have an intrinsic ability to splice themselves which is called self-splicing or auto splicing. Here no enzyme is required and RNA sequence functions as its own ribozyme and behaves like an endonuclease. In rRNA of Tetrahymena the splicing process involves binding of a single guanosine (G) to a site within the intron which is to be spliced out. Another ribozyme is ribonuclease P (RNAse P) which is a nucleoprotein ribozyme involved in the processing of pre-tRNA. The RNAse P is a ribozyme composed of one RNA component and one protein component. Its RNA component is a ribozyme which catalyzes the processing of precursors tRNA. Precursor tRNA is a very long molecule. During processing its large portion is removed and degraded. Precursor tRNA is cleaved at both 5′-end and 3′-end. In E. coli the tRNA which carries amino acid tyrosine has been extensively studied. The cleavage occurs differently at 5′-end and 3′- end. At the 5′-end the precursor tRNA is recognized by an enzyme known as RNAse P which is a ribozyme (as shown in diagram below). This ribozyme cleaves a portion of pre-tRNA so that correct 5′-end of mature tRNA is produced. This proves that all enzymes are not proteins. Ribozyme plays role in protein synthesis also, during elongation phase, a tRNA brings a new amino acid to the ribosome according to the codons of mRNA. Each new amino acid is then attached to the end of the growing polypeptide chain. During chain elongation, a peptide bond is formed between the amino acid at “A” site and growing polypeptide chain, which is transferred from “P” site to “A” site. The transfer is known as peptidyl transfer reaction. This transfer involves formation of peptide bond between amino acid at the “A” site and polypeptide chain, thus lengthening the chain by one amino acid. It was discovered that the peptidyl transferase which catalyzes the peptide bond formation between successive amino acids consists of several proteins and a 23S rRNA molecule present in the 50S subunit of ribosome. This 23S rRNA is a ribozyme and is responsible for catalyzing peptide bond formation between successive amino acid. Hammerhead Ribozyme: Hammerhead ribozyme is another small RNA which performs self- cleavage reaction. It is found in viroids, which have RNA as genetic material and they infect the plants. When viroid replicates by rolling circle, it produces a continuous RNA chain, which consists of multiple copies of RNA. This continuous chain RNA undergoes cleavage to form single viroids or monomers. The self-cleaving RNA sequence at the junction of monomers is called hammerhead because of the shape of its secondary structure. It consists of three complementary base paired stems. These are stem I, stem II and stem III surrounding a core of non-complementary nucleotides (as shown below). This core lies at their junction and undergoes self-cleavage reaction. The catalytic reaction centre lies between stem 2/3 and stem I. This center contains a magnesium ion that initiates hydrolysis reaction and breaks the RNA chain. Like antisense RNA, which is used to silence the genes, ribozyme is also used to turn off specific genes. Exon shuffling – Exon shuffling was first introduced in 1978 by Walter Gilbert. It is a molecular mechanism for the formation of new genes. That new gene may encode for mosaic (or chimeric) protein (a protein encoded by a gene that contains regions also found in other genes). The first described mosaic protein was tissue plasminogen activator. The existence of such proteins is evidence for exon shuffling. Exon shuffling is of three types – 1. Exon duplication = the duplication of one or more exons within a gene (internal duplication). 2. Exon insertion = exchange of domains between genes or insertions into a gene 3. Exon deletion = the removal of a segment from a gene. All types of shuffling have occurred in the evolutionary process of creating new genes. Note that in exon shuffling, it is DNA molecules that are being recombined, in contrast to alternative splicing, where different mature RNAs may be produced by combining different exons from the primary transcript. Exon shuffling can occur because of crossover during sexual recombination of parental genomes and there can be transposon mediated exon shuffling also. Exon shuffling could be represented through various phases as shown in diagram below – To understand this, we need to consider introns in terms of their possible positions relative to the coding regions. Introns residing between coding regions are classified into three types according to the way in which the coding region is interrupted. An intron is of phase 0 if it lies between two codons, of phase 1 if it lies between the first and second nucleotide of a codon, and of phase 2 if it lies between the second and third nucleotides of a codon. Exons are grouped into nine different classes (shown below) according to the phases of their flanking introns. An exon that is flanked by introns of the same phase at both ends is called a symmetrical exon (0-0, 1-1, 2-2), otherwise it is asymmetrical (0-1, 0-2, 1-0, 1-2, etc.). Numbers above the box represents the position of nucleotide .
Details
-
File Typepdf
-
Upload Time-
-
Content LanguagesEnglish
-
Upload UserAnonymous/Not logged-in
-
File Pages4 Page
-
File Size-