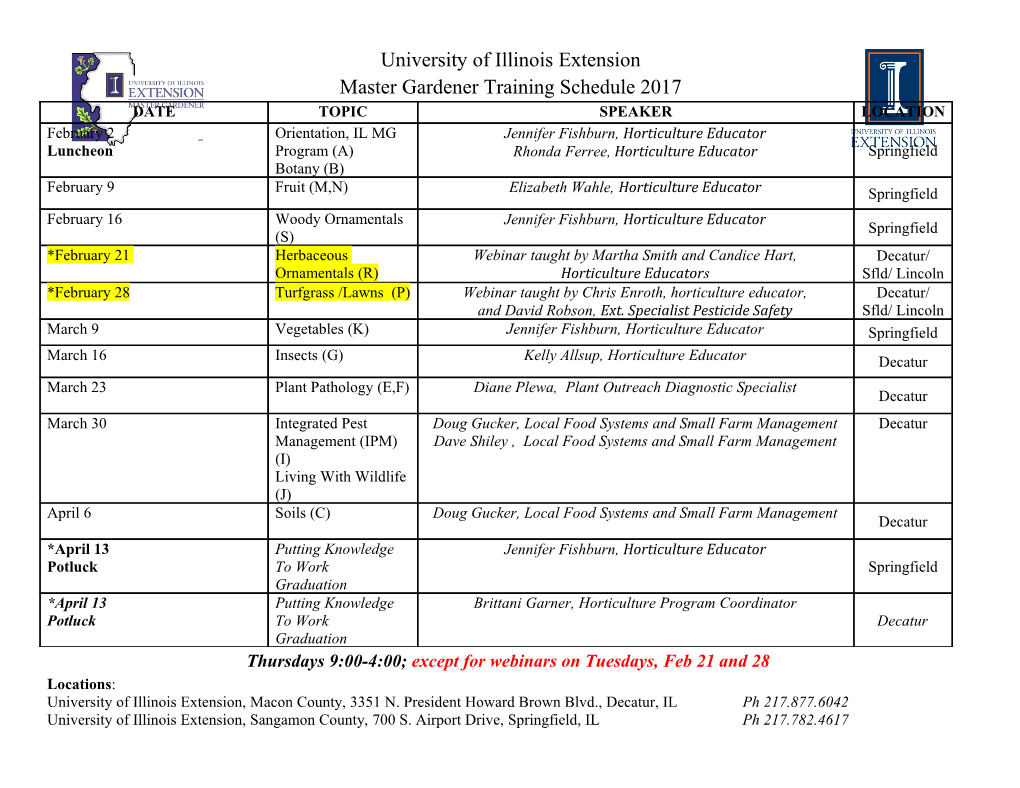
The Astrophystcal Journal, Vol. 150, December 1967 .150.1125M . J. Ap 67 19 THE UPPER ATMOSPHERE OF MARS* Michael B. McElroy Kitt Peak National Observatory,! Tucson, Arizona Received April 11, 1967 ABSTRACT A comprehensive model for the Martian upper atmosphere, consistent with results obtained in the recent Mariner IV occultation experiment, is developed. It is argued that the ionosphere detected in this experiment should not be interpreted as an F2 layer analogous to the terrestrial ionosphere. The possi- bility of an PI interpretation is explored, and attention is directed to important difficulties associated with this interpretation It cannot, however, be categorically excluded at this time. A detailed E region model is derived and shown to compare favorably with observation. The accuracy of thermal calculations is discussed. In particular it is argued that eddy transport does not provide an important mode of heat loss from the Martian thermosphere. It is concluded that 400° K represents a reasonable lower bound to the exospheric temperature on Mars. The photochemistry of CO2 is discussed briefly and attention is directed to the possible importance of reactions involving It is suggested that association of (X1/?) and CO may occur rapidly, and as a consequence the upper atmosphere may be deficient in dissociation products such as CO, O2, and 0. I. INTRODUCTION In the recent Mariner IV occultation experiment (Kliore, Cain, Levy, Eshleman, Fjeldbo, and Drake 1965), phase shifts of the telemetry carrier wave were measured on Earth as the spacecraft passed behind Mars. From the observed phase shifts it was pos- sible to infer densities and density gradients for the neutral atmosphere at low altitudes and, at high altitudes, similar information about ambient ionization. Derived values for surface pressure on Mars are in the range 4 to 7 millibars, and agree generally with recent ground based spectroscopic studies (Belton and Hunten 1966). The ionospheric measurements, however, revealed several surprises. A peak electron density of order 105 cm-3 was observed at an altitude of 125 km over Electris in early afternoon. The altitude of the observed ionization peak was unexpected, as was the small value observed for the scale height of electron density above the peak. We shall be concerned primarily in this paper with interpretation of the ionospheric measure- ments. The first papers (Kliore et al. 1965; Johnson 1965; Fjeldbo, Fjeldbo, and Eshleman 1966a) interpreting the Mariner data assumed that the ionospheric peak is of the F2 type. This interpretation was suggested by analogy with the terrestrial ionosphere where the ionization maximum is formed under the combined effects of diffusion and photo- chemistry. If the ionic mass is known, the observed scale height above the F2 peak gives the plasma temperature. It was assumed that 0+ is the dominant ion and a temperature of 85° K was derived for the Martian exosphere. Some arguments against an F2 interpretation were presented by Chamberlain and McElroy (1966). Their objections were twofold. First, the F2 models require extremely low particle densities (^ 109 cm-3) at 125 km. This result does not appear consistent with any available thermal calculations (as distinct from heuristic models) for the lower atmosphere. Furthermore, they argued on the basis of detailed thermal studies of the upper atmosphere that 400° K should represent a lower limit to the exospheric tempera- tures on Mars. Their model considered heating of the upper atmosphere by absorption of ultraviolet solar radiation and allowed for redistribution of energy by infrared radiation * Kitt Peak National Observatory Contribution No. 257. f Operated by the Association of Universities for Research in Astronomy, Inc., under contract with the National Science Foundation. 1125 © American Astronomical Society • Provided by the NASA Astrophysics Data System 1126 MICHAEL B. McELROY Vol. 150 and molecular conduction. A similar study has recently been published by Fjeldbo et al. (19666). We shall discuss thermal calculations of the upper atmosphere in § II and argue that the calculations of Fjeldbo et al., which yield low temperatures for the Martian exosphere, are suspect. Johnson (1967a) has drawn attention to the possible importance of eddy conductivity as a mechanism for heat loss in the Martian thermosphere. In his analysis the convective heat flux is derived from the coefficient of eddy diffusion. Here we dispute that this pro- cedure is correct and argue in § III that eddy transport of mass and energy are not re- lated in a simple way for Mars. In particular, mass mixing may occur to great heights on Mars without significant transport of heat. The objections to an F2 model for the Martian ionosphere depend primarily on calcu- lations of temperature profiles for the neutral gas. If the observations do indeed refer to an F2 layer, the topside scale height should reflect not the neutral temperature but a mean of electron and ion temperatures. At the F2 peak on Earth, the electron tempera- ture exceeds the gas temperature by a factor of about 2.5 (Evans 1965; Dalgarno, McElroy, and Walker 1967). If a similar situation prevailed on Mars, this would indicate neutral temperatures much less than values discussed earlier in connection with F2 models and would intensify already serious difficulties of reconciling F2 models with theory. The relationships between temperatures of electrons, positive ions, and neutral particles in the hypothetical Martian F2 layer are discussed in § IV. It will be shown that, unless electron heating rates on Mars are very much less than values obtained by scaling from Earth, the F2 model requires unacceptably small values for the neutral temperature. Alternative models for the Martian ionosphere are discussed in § V. Chamberlain and McElroy (1966) adopted a model for the lower atmosphere which contained appreciable amounts of N2 and, on the basis of computed number densities at 125 km, proposed an E region interpretation for the observed ionosphere. Donahue (1966a), however, specu- lated that the ionosphere might be consistent with an FI interpretation if the lower atmosphere contained essentially pure C02. Even in this extreme situation, we find that calculated number densities at 125 km favor an E rather than FI interpretation of the Mariner data. An illustrative E region theory is developed and shown to compare favorably with observation. The theory is consistent with recent laboratory data on important reaction rates. A major uncertainty in the model, however, concerns the rate for dissociative recombination of C02+, and we emphasize that measurements of this quantity are urgently required. II. THERMAL STUDIES OF THE UPPER ATMOSPHERE Pre-Mariner studies of the Martian upper atmosphere (Chamberlain 1962; McElroy, L’Écuyer, and Chamberlain 1965) indicated exospheric temperatures of order 700° K. These studies supposed C02 was dissociated in the thermosphere and did not therefore consider the possible importance of C02 as a thermostat. Chamberlain and McElroy (1966) allowed for this possibility and attempted to derive a lower limit to the exospheric temperature. Their result (400° K) is consistent with earlier studies but disagrees with recent calculations reported by Fjeldbo et al. (19666). We shall explore in this section possible reasons for the discrepancy. Our discussion will emphasize models with minimal dissociation; evidently models with significant dissociation are not consistent with the Mariner observations. In both calculations, the effects of eddy conductivity were ignored. Heat was supplied to the upper atmosphere by absorption of solar ultraviolet radiation, transferred by molecular conduction, and lost primarily by infrared radiations from C02. The tempera- ture was obtained by numerical integration of the heat equation d_ =o (i) dz 0 "^2) ^ ^ ^ e\<ri\h\e 5> - © American Astronomical Society • Provided by the NASA Astrophysics Data System .150.1125M No. 3, 1967 ATMOSPHERE OF MARS 1127 . J. where T denotes temperature, k thermal conductivity, ex the fraction of absorbed solar Ap energy deposited as local heat, <r*x the absorption cross-section for constituent i at 67 19 wavelength X, /0x the incident solar flux at this wavelength, fti the number density of constituent i, r\ an appropriate optical thickness, and Ri the energy radiated by con- stituent i. The optical thickness is defined by r00 y ^ rx = J sec xdz', (2) where x defines the relevant solar zenith angle. In equation one, the second term de- scribes solar heating of the atmosphere. It is assumed that on average the Sun is present half the time and x should represent a mean daytime solar zenith angle. Fjeldbo et al. (1966b) offered four reasons for the disagreement between their results and those reported by Chamberlain and McElroy (1966): 1. Their calculations were for the atmosphere above Electris whereas Chamberlain and McElroy considered a planetary mean. In practice, Chamberlain and McElroy adopted a value 60° for x whereas Fjeldbo et al. used 67°, the noon value for the solar zenith angle over Electris. We have verified that our calculations are not sensitive to a 7° change in solar zenith angle. 2. Radiative cooling was more efficient in their models because of a higher abundance of CO2. In arriving at a lower limit to the exospheric temperature, Chamberlain and McElroy investigated a wide range of possible chemical compositions in the Martian upper atmosphere. Some ten different models, including the limiting case of pure CO2, were considered. An increase in CO2 content did not result in any decrease of exospheric temperature since the atmosphere was already optically thick for 15-^ radiation. 3. Heat loss by conduction was more efficient in their models because of a higher abundance of 0. The effects of chemical composition on thermal conductivity were also explored by Chamberlain and McElroy, and the reported lower limit to exospheric temperature was not altered. 4. They used lower values for the quiet sunspot minimum solar flux below 200 Â. Intui- tively one does not expect exospheric temperature to depend sensitively on the assumed X-ray flux.
Details
-
File Typepdf
-
Upload Time-
-
Content LanguagesEnglish
-
Upload UserAnonymous/Not logged-in
-
File Pages14 Page
-
File Size-