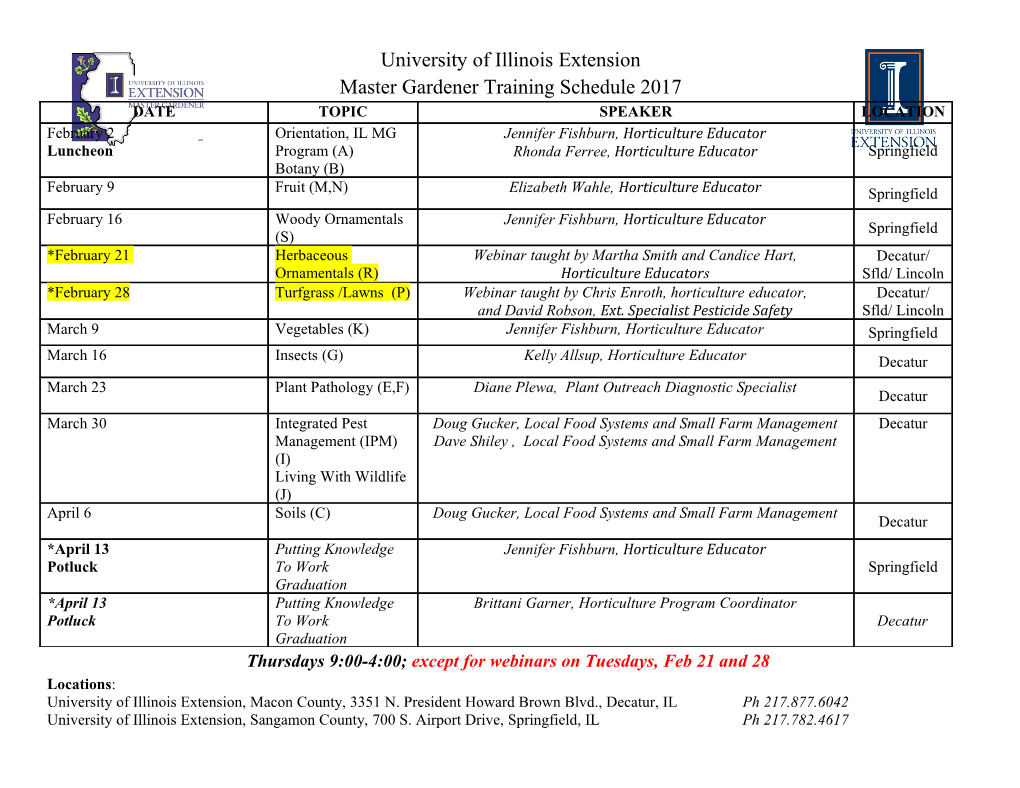
Challenge H: For an even safer and more secure railway Development of the Countermeasure against Roadbed Degradation under Ballastless Tracks for Existing Lines Katsumi MURAMOTO, Takahisa NAKAMURA Track Structures and Geotechnology Laboratory, Railway Technical Research Institute, Tokyo, Japan Recently, ballastless tracks with cement grouted into ballast have been constructed on a full-scale construction operation in Japan Metropolitan Area. If the ballastless tracks are laid on clayey roadbeds, the soils are likely to be fluidized and flow out by trainloads. Consequently, track degradations typified by a large track depression sometimes occur. In this study, the authors carried out the test with a full-scale ballastless track model laid on a saturated clayey roadbed. From the results of the test, it was confirmed that degradation of the ballastless track on a clayey roadbed is caused by local progressive failure; specifically, the outflow of the fine-grain fraction in roadbed surface. In addition, the authors confirmed that the BLITS (Bentonite Liner for Track-bed Surface) method is one of the reasonable countermeasures for the roadbed degradation under the ballastless tracks. Keywords: roadbed, ballastless track, roadbed degradation, mud pumping, bentonite 1. Introduction When ballastless tracks (Fig. 1) are constructed on sound roadbeds, the maintenance work and the maintenance costs become generally less than conventional ballast tracks. However, if the ballastless tracks are laid on clayey roadbeds, the soils are likely to be fluidized and flow out by the trainload. Consequently, track degradations typified by a large track depression sometimes occur. The authors, therefore, have carried out moving-load tests with small-scale ballastless track models on clayey roadbeds and reappearance tests of the roadbed degradation using small-scale cylindrical molds, so that the basic defect mechanism of clayey roadbeds has been clarified (Fig. 2).1) Accordingly, three fundamental policies of countermeasures against clayey roadbed defects were clarified as follows2): 1) Decrease a dynamic water pressure on the roadbed surface, which is excited by trainload 2) Decrease roadbed pore water level 3) Increase roadbed soil cohesion The results of small-scale model tests, however, might not be applicable to actual conditions because the phenomenon involving pore water movement differs significantly with the effect of scale. Furthermore, the progressive failures involving roadbed soil outflow are hardly predictable using numerical simulation at 1 this time. In this study, we performed tests with full-scale track models to clarify the details of roadbed degradation and countermeasure effectiveness. Rail PC-Sleeper Cement Grouted Layer Ballast Penetration Ballast Ground Side Ditch Fig. 1 Ballastless Track for Existing Lines Train Load Outflow of mud Inflow of rain water Cave Formation Water Channel Fig. 2 Basic Defect Mechanism of Clayey Roadbeds 2. Simulation of the roadbed degradation process by full-scale model tests 2.1 Setup of full-scale clayey roadbed model Where a full-scale roadbed model is made with clayey soil, the roadbed is, generally, constructed by two methods; compaction with the soil, which is controlled by optimal water content; consolidation with slurry, which is controlled by higher water content than the liquid limit. Because roadbed saturation and loading history were more important in these tests, the models were made by consolidation. Fig. 3 shows an outline of the construction process of the full-scale roadbed model. The clay slurry was pumped into an earth tank (Fig. 4), which was then depressurized after the roadbed surface had been covered with a polyethylene film. The differential stress between the internal pressure and atmospheric pressure acts on the roadbed surface and squeezes pore water from the slurry. As a result, a saturated clayey roadbed with a controlled stress history is constructed, namely by the vacuum consolidation method. 2 Polyethylene Film Air-Water Separation Layer Supernatant Water (Plastic Pallet) Clay Slurry Sand Filter (Nonwoven Cloth) Layer 1) Cast in slurry and settle out 2) Set plastic pallet and polyethylene film to Vacuum Pump Vacuuming Atmosphere Pressure Atmosphere Pressure Ballastless Track Model Water Trap Clay Slurry Clayey Roadbed Drain Pipe Vacuuming 3) Vacuum and consolidate 4) Set track model Fig. 3 Making Procedures of Roadbed Table 1 Properties of Arakida clay Particle Density 2.712g/cm3 Liquid Limit 49.4% Plastic Limit 27.7% Plasticity Index 21.7 Rate of Sand Content 2.7% Rate of Silt Content 50.6% Rate of Clay Content 46.7% Fig. 4 Slurry Casting 2.2 Model outline The properties of Arakida–clay, which was used in this experiment, were as shown in Table 1. This clay is derived from volcanic cohesive soil and distributed around the Arakawa (a river in Japan’s Kanto region). Although Arakida–clay is well known as a good compaction soil and used for playing fields, we had already clarified that this clay easily causes degradation under trainloads3). Fig. 5 and Fig. 6 show the outlines of the model. The slurry was formed into a clayey roadbed approximately 700 mm thick by the vacuum consolidation method. Ballast penetration and concrete plates that simulate irregularities at the bottom of the grouted layer were buried in the roadbed surface. In addition, pore pressure meters were set near these buried items. In substitution for the ballastless track for existing lines, an A-type Shinkansen concrete slab was used 3 for the model in this experiment because the slab width is practically equal to that of a cement grouted layer of the ballastless track. Besides, it had been confirmed by FE analysis that bending rigidity of the slab is also almost equal to the ballastless track (Fig.7)4). Ballast Penetration Pore Water Pressure Meter A-Type Concrete Slab for Shinkansen Concrete Plate 3000mm(5 fastenings) 3000mm(5 Concrete Plate Ballast Pore Water Penetration Pressure Layer Meter Depth=700mm 2340mm Fig. 5 Cross Section of the Model Fig. 6 Roadbed Surface Wheel Load = 50kN Track Structure Model 0.0 Loading Actuator Ballastless Track for Exisiting Lines A-Type Slab Track for Shinkansen 0.5 Roadbed Displacement 1.0 Slab Vertical Displacement (mm) Displacement Vertical Rail Displacement 1.5 0 500 1000 1500 Distance from Center of the Model (mm) Fig. 7 Result of FE Analysis Fig. 8 Situation of the Cyclic Loading 2.3 Test specifications Fig. 8 shows a situation of the cyclic loading. The loading points were the center of the rails with five rail fasteners on the slab. The cyclic load, which was a 0 to 100kN sine wave, was acted on the loading points with a 1-Hz frequency. This 1-Hz frequency was determined from the predominant frequency of the dynamic water pressure that was measured under an actual ballastless track on a conventional (non-Shinkansen) line (Fig.9). This frequency depends on car length; therefore, eighty thousand times correspond to the monthly operation of a busy line in Japan. The roadbed settlements under ballastless tracks on operation lines must be approximately finished because sufficient loading history was applicable under the operation with ballast tracks. Therefore, the maximum consolidation pressure was set at 80kPa because the model roadbed has to be over 4 consolidation condition. In addition, static preloading, in which a drainage layer is put on the roadbed, was imposed with a 300kN axle load for 24 hours before a cyclic loading test with a 100kN axle load. Fig. 10 shows an outline of the loading history. 2 Water Pressure of the Roadbed Surface 1 0 ) Consolidation a 80 P Pressure k ( -1 (80kPa) Car Length e r u Bogie to Bogie 1.0 s s Preloading e r Water(kPa) Pressure -2 0.5 (Axle Load = 300kN) P d 46 Cyclic Loading Test 0.0 e b (Axle Load = 100kN) d -3 -0.5 a o R Roadbed Displacement -1.0 18 n a Displacement(mm) -4 -1.5 e 0 2 4 6 8 10 12 14 16 18 20 M 4 Time (sec) Self Weight of The Slab Approximately 3 ton Fig. 9 Water Pressure under Ballastless Track Fig. 10 Outline of Loading History 2.4 Observation of the degradation Fig. 11 shows the primitive phase of the degradation, when muddy water with a high water content moves in and out through water channels that have developed around the ballast penetration layers or the concrete plates. Then, as shown in Fig. 12, silty soil, which has lower water content than the primitive phase muddy water, is pushed out from the water channels and accumulates on the roadbed at the terminal phase of degradation. Fig. 13 shows the roadbed surface when the concrete slab was removed after the loading test. Emanating from the ballast penetration layers, ramal channels filled with soft mud arose on the roadbed surface. The entire roadbed surface was thinly covered with soft mud. The relationship between number of times under load and track settlement, in other words rail settlement, is shown in Fig. 14. The track settlement increased by 4 mm to 5 mm at a stretch until primal thousands times under loading. It was assumed that this primitive settlement occurred because the roadbed surface became softer and weakened by the cyclic water pressure. The primitive settlement corresponded to the primitive outflow of muddy water shown in Fig. 11. The settlement rate of the track was reduced immediately after the primitive settlement, however that eventually accelerated by degrees. It was assumed that this acceleration occurred due to spreading of the water channels over the roadbed surface (Fig. 13) and flowing out of silty soil which has less viscosity with little clay content (Fig. 12). Fig. 15 shows the relationship between number of times under load and amplitude of the track displacement. The amplitude increases at a stretch until primal thousands times under loading and then increases at a constant rate.
Details
-
File Typepdf
-
Upload Time-
-
Content LanguagesEnglish
-
Upload UserAnonymous/Not logged-in
-
File Pages10 Page
-
File Size-