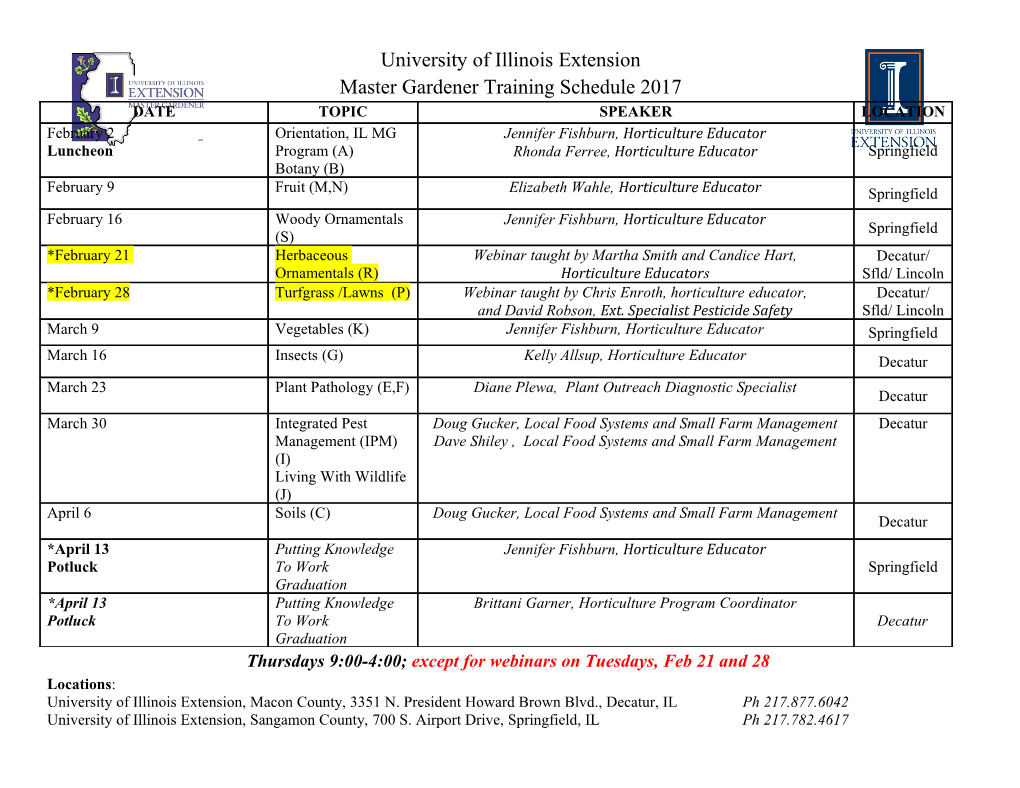
High mass diffraction at the LHC Christophe Royon DAPNIA-SPP, CEA Saclay, Fermilab, Batavia, USA DIS 2005, April 26- May 1 2005, Madison Work done in collaboration with J. Cammin (Rochester), R. Peschanski, M. Boonekamp, S. Lavignac (Saclay), A. Kupco (Prague) Ref: hep-ph/0406061, hep-ph/0504199 Contents: • Exclusive standard model and SUSY Higgs production: S/B • W, top and stop production cross section • W, top and stop mass reconstruction 1 “Exclusive models” p p P g k 1 g k 1 x x , γγ 1 H , QQ , γγ 1 H , QQ g x k 2 g k 2 x 2 2 P p "Standard" p "Exclusive " p P g k x 1 1 H , QQ , γγ g x k 2 2 P p "Inclusive" All the energy is used to produce the Higgs (or the dijets), namely xG ∼ δ 2 Advantage of exclusive Higgs production? • Good Higgs mass reconstruction: fully constrained system, Higgs mass reconstructed using both tagged protons in the final state (pp → pHp) • MH = pξpξp¯S • No energy loss in pomeron “remnants” 14000 Entries 22777 12000 10000 8000 6000 4000 2000 0 0 20 40 60 80 100 120 140 160 180 200 Mx 8000 Entries 22777 7000 6000 5000 4000 3000 2000 1000 0 118 118.5 119 119.5 120 120.5 121 121.5 122 Mx 3 DPEMC Monte Carlo • DPEMC (Double Pomeron Exchange Monte Carlo): New generator to produce events with double pomeron exchange http://boonekam.home.cern.ch/boonekam /dpemc.htm, hep-ph/0312273 • Interface with Herwig: for hadronisation • Exclusive and inclusive processes included: Higgs, dijets, diphotons, dileptons, SUSY, QED, Z, W ... • DPEMC generator interfaced with a fast simulation of LHC detector (as an example CMS, same for ATLAS), and a detailled simulation of roman pot acceptance 4 “Exclusive” production at the LHC • Higgs decaying into b¯b: study S/B • Exclusive b¯b cross section (for jets with pT > 25 GeV): 2.1 pb • Exclusive Higgs production (in fb) MHiggs σ (fb) 120 3.9 125 3.5 130 3.1 135 2.5 140 2.0 • NB: a survival probability of 0.03 was applied to all cross sections 5 LHC: where to put roman pot detectors 3 different options to implement roman pot detectors • (1) 215 meter pots (warm section) in addition to Totem |t| < 2 GeV2, 0.02 <ξ< 0.2, can be introduced in trigger and for luminosity determination • (2) 308-336 meter pots (cold section) |t| < 2 GeV2, 0.003 <ξ< 0.025 difficult technically • (3) 420 meter pots |t| < 2 GeV2, 0.002 <ξ< 0.016 • In the following, we keep options (2) and (3) together 6 LHC: roman pot acceptance Acceptance as a function of Higgs mass for different roman pot configurations 7 Signal and background Signal and background for different Higgs masses for 100 fb−1 35 40 35 30 30 25 25 20 20 15 15 10 10 5 5 0 0 100 105 110 115 120 125 130 135 140 145 150 100 105 110 115 120 125 130 135 140 145 150 total mass pots total mass pots 30 25 25 20 20 15 15 10 10 5 5 0 0 110 115 120 125 130 135 140 145 150 155 160 120 125 130 135 140 145 150 155 160 165 170 total mass pots total mass pots 8 Signal over background: standard model Higgs For a Higgs mass of 120 GeV and for different mass windows as a function of the Higgs mass resolution S/B 10 9 8 7 6 5 4 3 2 1 0.9 0.8 0.7 0.6 0.5 0.4 0.3 0.2 0 1 2 3 4 5 Mass resolution 9 Signal over background Signal over background for 1 mass window (2 GeV wide) for 100 fb−1 assuming a Higgs mass resolution of 1 GeV MHiggs signal background S/B σ 120 27.1 28.5 0.95 5.1 130 20.6 18.8 1.10 4.8 140 12.6 11.7 1.08 3.7 150 7.0 8.9 0.69 2.3 NB: if only tags at 420 m, numbers have to be divided by about 50% 10 Diffractive SUSY Higgs production High tan β: top and bottom loops to be considered, enhance the cross section by up to a factor 50 (worth looking into Higgs decaying into b¯b since branching ratio of Higgs decaying into γγ smaller at high tan β, standard search in γγ does not benefit from the increase of cross section) correction factor vs m h µ 30 = -200 Gev mSUSY = 300 GeV factor 25 tanβ = 30 20 maximal mixing minimal mixing 15 10 5 0 90 95 100 105 110 115 120 mh, GeV correction factor vs tanβ µ = -200 Gev 60 mSUSY = 300 GeV factor mA = 100 GeV 50 40 maximal mixing minimal mixing 30 20 10 0 0 10 20 30 40 50 tanβ 11 W, top and stops p p Pom γ 1 g W W g t t g γ Pom p p All the energy is used to produce the W, top (stop) pairs: W: QED process, cross section perfectly known, top: QCD diffractive process 12 Top and W events 9 12 8 Events Events 10 7 6 8 5 6 4 3 4 2 2 1 0 0 140 150 160 170 180 190 200 210 220 300 320 340 360 380 400 420 440 460 480 500 total pot mass (GeV) total pot mass (GeV) • W boson cross section and acceptance: σ ∼ 56 fb, pots at 420 m needed, about 60% • Top quark cross section and acceptance: σ ∼ 40 fb, pots at 220 m, about 85% • Reconstruct the W and top mass using the threshold scan method: Fit the increase of the cross section at threshold 13 Resolution on W and top masses 2.4 1.4 res = 3.0 GeV 2.2 res = 3.0 GeV (GeV) (GeV) t W res = 2.0 GeV res = 2.0 GeV 1.2 2 res = 1.0 GeV res = 1.0 GeV 1 1.8 1.6 0.8 1.4 0.6 1.2 0.4 1 0.8 0.2 Expected stat. uncertainty m Expected stat. uncertainty m 0.6 0 100 200 300 400 500 600 50 100 150 200 250 300 350 400 Integrated luminosity (fb-1) Integrated luminosity (fb-1 • 2 methods uaed to reconstruct the top mass: histogram: (compute χ2 between number of events in bins in MC and data for the same lumi), turn-on fit: fit the turn-on point of the missing mass distribution at threshold • W mass resolution: ∼ 400 MeV, not competitive, but allows to calibrate (align) roman pots very precisely • Top mass resolution: ∼ 1 GeV, competitive measurement 14 Top and stops • Cross section for a stop mass of 250 GeV: σtot = 8 fb, σacc =6 fb • Possibility to distinguish between top and stop even if they have about the same mass: using the differences in spin (as an example: mt˜ = mtop) • Very fast turn-on for stops 100 80 60 40 20 0 300 350 400 450 500 550 600 total mass pots 15 Stop production cross section • Stop production cross section as a function of the stop mass: quite high cross section (1 fb for a stop mass of 400 GeV) • Numbers be multiplied by the roman pot acceptance of about 80% 2 cross (fb) 10 10 1 -1 10 100 150 200 250 300 350 400 450 500 M stop 16 Resolution on stop mass Resolution on stop mass by using roman pot detectors with a resolution of 1 GeV → Resolution better than 1 GeV at high lumi! 1.4 m~ = 210 GeV t (GeV) t ~ 1.2 1 0.8 0.6 m~ = 174.3 GeV t 0.4 Expected stat. uncertainty m 0.2 0 40 60 80 100 120 140 160 180 200 Integrated luminosity (fb-1) 17 Conclusion • Study of exclusive Higgs production • Exclusive events still to be observed in particular at the Tevatron • Signal over background: ∼ 1 if one gets a very good resolution using roman pots (better than 1 GeV), enhanced by a factor up to 50 for SUSY Higgs at high tanβ • QED WW pair production: cross section known precisely, allow to calibrate prescisely the roman pot detectors • Diffractive top, stop pair production: possibility to measure top and stop masses by performing a threshold scan with a precision better than 1 GeV (same idea as linear collider, without ISR problem), Caveat: evidence of exclsuive events, cross sections???... 18.
Details
-
File Typepdf
-
Upload Time-
-
Content LanguagesEnglish
-
Upload UserAnonymous/Not logged-in
-
File Pages18 Page
-
File Size-