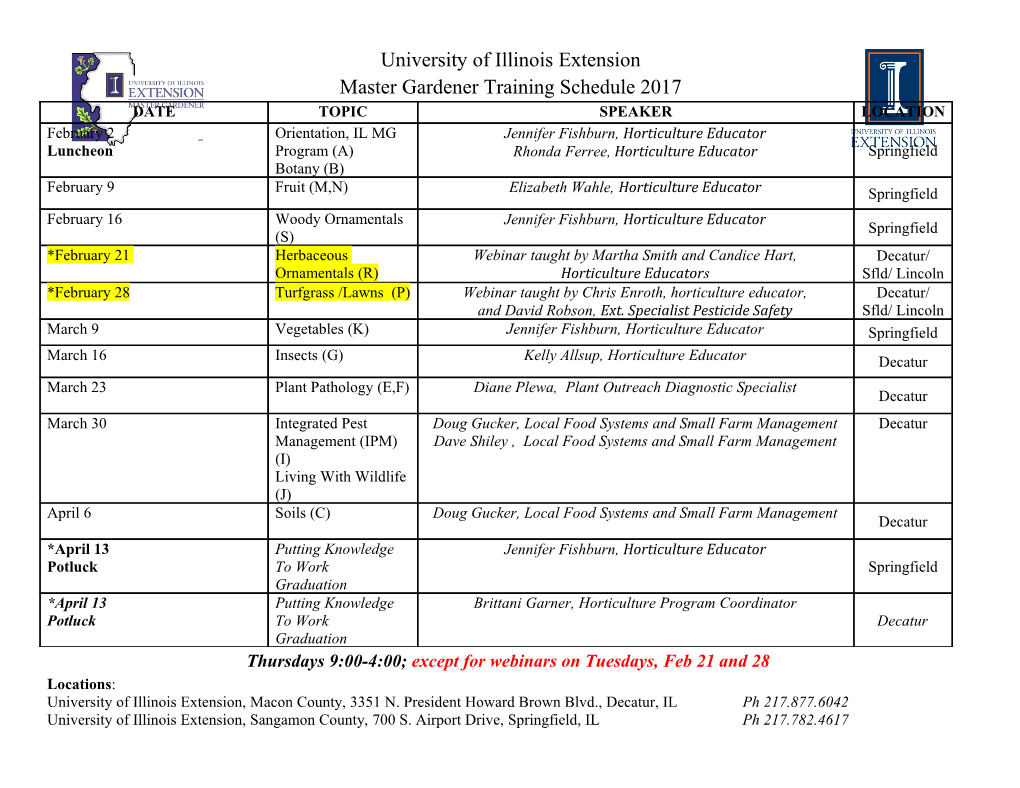
The fabrication of arrays of single ions in silicon via ion implantation Jessica van Donkelaar Submitted in total fulfillment of the requirements of the degree of Doctor of Philosophy February 2014 School of Physics, Faculty of Science The University of Melbourne Abstract Conventional computers are approaching fabrication limits where statistical variation in the placement of single dopants greatly impacts device perfor- mance. Additionally, practical modelling of more than a handful of atoms while accounting for quantum properties can only be performed with a quan- tum computer. Fabricating a solid state Si:P Kane architecture will require deterministic doping; the ability to accurately place dopants within a sub- strate. We have implemented an apparatus for creating arrays of single P donor atoms within Si. We model the fabrication of a three-donor device for demonstrat- ing the coherent transfer by adiabatic passage transfer (CTAP) protocol and show that it can be done with deterministic ion implantation through a 20nm nanostencil stepped 30 nm between implants to produce a yield of 1-in-7 well placed arrays. Single ion implantation is an established method of device fabrication that involves detecting single 14 keV P+ ions entering a substrate via the charge induced as the ion collides with the lattice. We have tested various designs for the single ion detector to evaluate the sources of good performance and achieved 98 4 % counting efficiency with the classic design in high purity sil- ± icon. We have fabricated 200 nm think Si3N4 nanostencils with slot and hole shaped apertures as small as 20 nm using the focusssed ion beam (FIB) technique of 0.0.0 back filling larger apertures with Pt. These adequately mask a 14 keV P+ ion to define the implant site. We have built apparatus that employs a piezo-positioner to move the nanos- tencil above the surface of a single ion detector. This system is LN2 cooled within a high vacuum to implement deterministic doping. The fine alignment of the nanostencil to the implant site is performed using the ion impact signals as feedback over a patterned area of the detector and we have demonstrated this concept to a precision of sub-100 nm. Declaration This is to certify that: 1. The thesis comprises only my original work towards the PhD except where indicated. 2. Due acknowledgment has been made in the text to all other material used. 3. The thesis is less than 100,000 words in length, exclusive of tables, bib- liographies, appendices and footnotes. Jessica van Donkelaar Preface The Si substrate detectors we employ for single ion implantation are fabri- cated at the Semiconductor Nanofabrication Facility (SNF) at the University of New South Wales (UNSW) as a collaboration between nodes of the Centre for Quantum Computer Technology (CQCT). The detectors produced during the course of this work in particular were fabricated primarily by Nai Shyan Lai and Fay Hudson. Post doctoral fellow Andrew Alves collaborated in designing the apparatus and worked with me to get the MeV IBIC data of section 3.0.9 and the shadow mask implants of section 5.2. Acknowledgments I would like to thank the many people who provided both technical assistance and moral support during my PhD project. My sincere thanks go to Roland Szymanski for showing me the inner workings of the pelletron and to Alberto Cimmino similarly laying bare the colutron. I am grateful to Sergey Rubanov for the training of the FIB/SEM system and assistance with the TEM. Thank you Andy Greentree for CTAP advice, to Changyi Yang for all his invaluable help in the lab and to Andrew Alves for working with me. Thank you to my supervisor David Jamieson for his enthusiasm and attentive guidance over the years. And finally, thanks go to my family and friends for their support, especially my parents Meryl & Patrick van Donkelaar and my husband David Perry. Contents 1 Introduction 1 1.1 History of the transistor; physics and fabrication . 3 1.1.1 Currentstateofthetransistorart . 9 1.2 QuantumComputing. 12 1.2.1 QCschemes......................... 14 1.2.2 CoherentTransport. 25 1.3 DeterministicDoping . 28 2 Modelling Ion Implantation 39 2.1 Ion-solidinteraction. 39 2.1.1 Electronicstopping . 44 2.1.2 Nuclearstopping ...................... 47 2.1.3 Binary Monte-Carlo simulation . 50 2.2 Coherent tunneling by adiabatic passage . 55 2.3 Modelling .............................. 59 3 Single ion implantation 70 3.0.1 The detector device . 75 3.0.2 Fabrication ......................... 79 3.0.3 Operation .......................... 81 3.0.4 Pulseheight......................... 84 3.0.5 Noise............................. 86 ii 3.0.6 Resolution.......................... 90 3.0.7 Newdetectordesigns . 92 3.0.8 Performanceresults. 94 3.0.9 MeV IBIC analysis . 101 3.0.10 Summary ..........................106 4 Constructing a nanostencil 109 4.1 Available fabrication methods . 110 4.2 TheFIBsystem...........................117 4.2.1 Beamdiameter . .118 4.2.2 Deposition resolution . 120 4.3 Results................................124 4.4 Summary ..............................131 5 Deterministic Doping 132 5.1 Theuseofananostencil . .132 5.2 Timed shadow mask implants through a multi-step nanostencil . 137 5.2.1 Results............................139 5.2.2 Lifetime of the nanostencil . 143 5.3 DeterministicDoping . .143 5.3.1 Alignment of the aperture to the implant site . 146 5.3.2 MeVnanoIBIC . .150 5.3.3 14 keV nanoIBIC . 155 5.3.4 Summary ..........................160 6 Conclusions 164 iii List of Figures 1.1 Band diagram for crystalline Si. 4 1.2 Theprogressionoftransistorstructure. 10 1.3 Spinqubitrepresentations.. 13 1.4 Depiction of NMR spin qubits (a) molecules suspended in liquid and(b)Sisolidstate......................... 15 1.5 Depiction of (a) trapped ion and (b) trapped atom qubit devices. 17 1.6 Images of superconducting (a) charge, (b) flux and (c) phase qubitdevices. ............................ 19 1.7 Images of physically defined quantum dot structures made with (a) Si:P, (b) cavity QED and (c) heterostructures. 20 1.8 Images of electrostatic quantum dot qubit devices in (a) GaAs/AlGaAs, (b)Si/SiGeandc)SiMOS. 21 1.9 Spin transport techniques including (a) CNOT swapping, (b) CTAPand(c)spinbus. ...................... 26 1.10 The bottom-up STM process of placing P atoms on to Si. 29 1.11 Methods of deterministic ion implantation. 31 1.12 Thedeterministicdopingapparatus. 34 1.13 A schematic of the deterministic doping process. 35 1.14 Comparison of the electrical characteristics of ordered donor arrays to random dopant placement on a 100 nm scale (a) and 10nmscale(b). ........................... 37 1.15 The dopant structures made possible by deterministic doping. 38 iv 2.1 Comparison of SRIM modelling to Geant4 modelling in the low energyheavyionregime. 42 2.2 Schematic of the products resulting from ion-solid interaction. 44 2.3 Stopping force comparison of 0.5 MeV He+ and 14 keV P+. 51 2.4 The three donor proof-of-concept device for demonstrating CTAP. 55 2.5 Depiction of various systems which utilise the (a) STIRAP and (b)CTAPtransportmechanisms. 57 2.6 The (a) alternating and (b) straddling CTAP schemes for long rangeCTAPtransport.. 58 2.7 Considerations for fabricating the CTAP device with ion im- plantation (a)(b) spacings and (c)(d) ion straggle. 60 2.8 The CTAP tunnel matrix elements of two donor placement sce- narios that demonstrates the effect of fabrication limitations. 62 2.9 Modelling results that give the fraction of well placed donors for CTAP when fabricated by ion implantation. 67 3.1 A schematic of the deterministic doping process. 71 3.2 (a) Schematic of the Colutron 14kV ion accelerator, (b) the ion source, (c) Fischer slits and (d) the target chamber. 72 3.3 The band bending of p-n junctions that determines charge car- rierflow................................ 76 3.4 A schematic of the PiN detector device from (a) the side and (b)above. .............................. 80 3.5 Thebasicpreamplifiercircuit. 82 3.6 Leakage current under reverse bias. 83 3.7 Signal sorting to obtain a pulse height spectrum. 84 3.9 Design schematics of the detectors studied in this chapter (a) DP,(b)DP-CS-Band(c)DP-CS-C. 95 3.10 Reverse bias breakdown (a) theory and (b)(c) measured in our PiNdetectors............................. 99 v 3.11 Comparison of dark current of a good, stable detector and a poordetector. ............................100 3.12 Schematic of the Pelletron 5MU accelerator. 103 3.13 IBIC CCE image of a single ion detector obtained by Changyi Yangin2006. ............................104 3.14 IBIC CCE map of a DP-CS-B detector. 104 3.15 IBIC CCE map of a DP-CS-C detector. 105 4.1 Depiction of lithography methods. 111 4.2 Comparison of the depth and physical distribution of UV pho- tons, high energy protons and low energy heavy ions used in lithography. .............................113 4.3 The scattering of 10 keV electrons. 114 4.4 Schematic of the FEI Nova 600 Nanolab dual FIB/SEM system used for the fabrication of nanostencils. 118 4.5 Schematic of electron and ion focussing within an SEM and FIB columnrespectively.. .119 4.6 Depiction of the Pt deposition process as in [1]. 122 4.7 Deposition profiles obtained at different current to precursor ratios. ................................123 4.8 Various SEM images of issues encountered during the FIB fab- ricationprocess.. .125 4.9 (a) the current feedback during the inital FIB milling and (b) images showing Pt deposition narrowing the initial aperture. 127 4.10 TEM images of the FIB milled slots before Pt deposition. 129 4.11 TEM images of 20 nm wide (a) slot and (b) hole apertures . 130 5.1 Comparison of shadow mask techniques for metal evaporation andionimplantation.. .134 vi 5.2 Depiction of ion scattering through the mask regions surround- ingananostencilaperture. .137 5.3 Images of (a) alignment markers on the sample and (b) the nanostencil used for the experiment.
Details
-
File Typepdf
-
Upload Time-
-
Content LanguagesEnglish
-
Upload UserAnonymous/Not logged-in
-
File Pages245 Page
-
File Size-