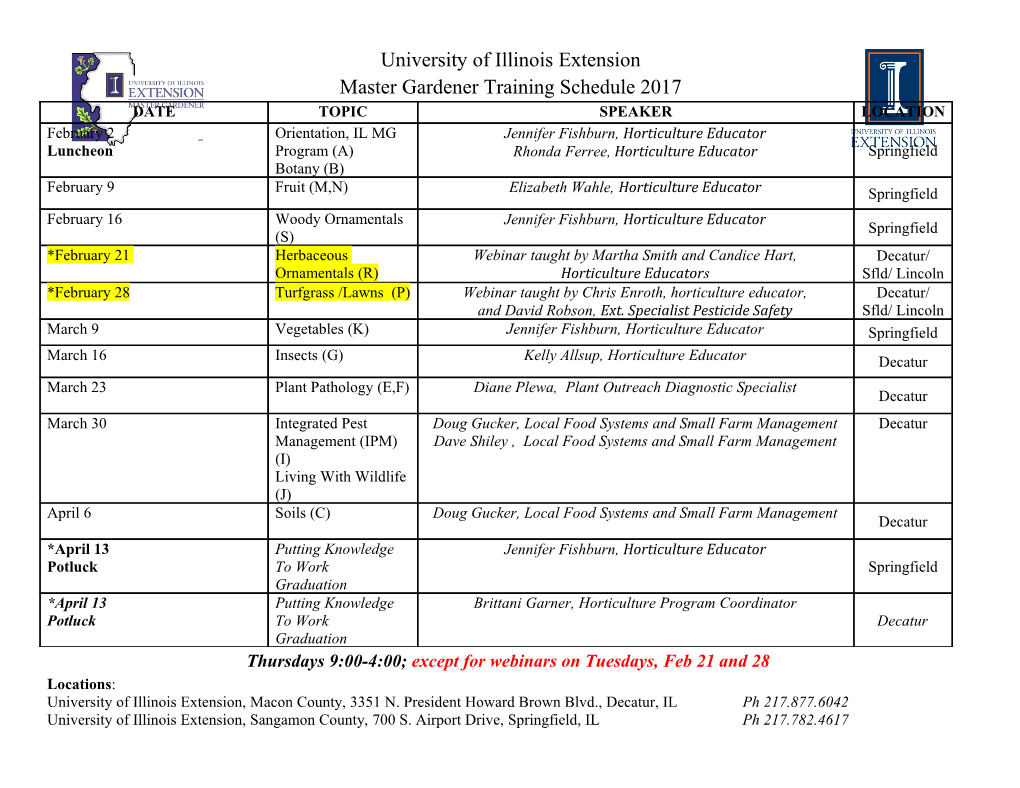
ARTICLE Received 3 Apr 2013 | Accepted 17 Jun 2013 | Published 16 Jul 2013 DOI: 10.1038/ncomms3155 A hybrid high-speed atomic force–optical microscope for visualizing single membrane proteins on eukaryotic cells Adai Colom1,*, Ignacio Casuso1,*, Felix Rico1 & Simon Scheuring1 High-speed atomic force microscopy is a powerful tool for studying structure and dynamics of proteins. So far, however, high-speed atomic force microscopy was restricted to well-con- trolled molecular systems of purified proteins. Here we integrate an optical microscopy path into high-speed atomic force microscopy, allowing bright field and fluorescence microscopy, without loss of high-speed atomic force microscopy performance. This hybrid high-speed atomic force microscopy/optical microscopy setup allows positioning of the high-speed atomic force microscopy tip with high spatial precision on an optically identified zone of interest on cells. We present movies at 960 ms per frame displaying aquaporin-0 array and single molecule dynamics in the plasma membrane of intact eye lens cells. This hybrid setup allows high-speed atomic force microscopy imaging on cells about 1,000 times faster than conventional atomic force microscopy/optical microscopy setups, and allows first time visualization of unlabelled membrane proteins on a eukaryotic cell under physiological conditions. This development advances high-speed atomic force microscopy from molecular to cell biology to analyse cellular processes at the membrane such as signalling, infection, transport and diffusion. 1 U1006 INSERM, Universite´ Aix-Marseille, Parc Scientifique et Technologique de Luminy, 163 avenue de Luminy, 13009 Marseille, France. * These authors have equally contributed to this work. Correspondence and requests for materials should be addressed to S.S. (email: [email protected]). NATURE COMMUNICATIONS | 4:2155 | DOI: 10.1038/ncomms3155 | www.nature.com/naturecommunications 1 & 2013 Macmillan Publishers Limited. All rights reserved. ARTICLE NATURE COMMUNICATIONS | DOI: 10.1038/ncomms3155 igh-speed atomic force microscopy (HS-AFM)1 has been of the AFM cantilever detection (Fig. 1, red arrows) from the proven to be a unique and powerful tool for the visible light OM signals (as will be detailed below). We removed Hconcomitant analysis of the structure and dynamics of the l/4 plate and the polarization beam splitter in the optical path single biomolecules2. HS-AFM was used to visualize myosin-V that was initially designed to separate the incident and the 3 4 walking , cellulase cellulose degradation ,F1-ATPase rotary reflected laser beams to and from the cantilever, respectively. The catalysis5, bacteriorhodopsin photocycle6, and OmpF diffusion polarization beam splitter has been replaced by a 45° mirror that and interaction7. However, HS-AFM has been restricted so far to deflects only the reflected laser beam. Indeed, we physically well-controlled molecular systems of purified proteins under well- separated the incident and the reflected laser beams by about controlled conditions. These systems were characterized by a 1 mm by displacing the incident laser by 0.5 mm with respect to limited number of pure molecular species with small corrugation, the centre position of the objective that focuses the laser onto the mainly because the fast z-piezo that follows the topography backside of the cantilever. The mirror directs the entire reflected profile has a small extension range (typically 400 nm; ref. 8). laser beam onto the photo detector split diode, while the beam Recently, the development of a wide-range HS-AFM scanner splitter did not reflect all the light and part of the beam was allowed the first nanoscale observation of molecular movements transmitted into the SLD potentially creating optical feedback. at two frames per second on living bacteria9. This alteration resulted in a higher signal-to-noise ratio and Historically, AFM debuted as early as 1990 for cell imaging hence more sensitivity of the deflection detection. Further major applications10, prompted by the need to perform cell structural changes/integrations to build the OM path are the following: in analysis at a resolution superior to light microscopy. However, the heart of the setup, we integrated a low-pass (o700 nm) AFM images of cells revealed rather the cell interior, like actin dichroic mirror (Fig. 1, label 4), allowing recovery of the AFM filaments, than the membrane structure of the cells. Later, AFM SLD750 nm beam and the passage of optical signals below 700 nm; and optical microscopy (OM) were combined in order to take a fluorescence light source (Fig. 1, label 2) injects light through a advantage of OM’s large-scale overview imaging capacities and its fluorescence filters cube (Fig. 1, label 6) that allows the tuning of power to analyse fluorescence signal targets of proteins of the wavelength of the incident light for excitation of the sample- interest11. For this purpose, AFMs were built in a table-top specific fluorophore (Fig. 1, blue arrow). The fluorescence filters configuration and mounted on inverted optical microscopes12.In cube is identical to the ones used in conventional fluorescence order to avoid modifications of the conventional inverted OM, microscopes and allows the same versatility for fluorescence two types of table-top AFM configurations were built. In one of experiments. The cube is located outside of the hard body of the them, the AFM tip and the scanner is combined into a single HS-AFM allowing easy exchange of the filters for the fluorophore moving component, and in the other a large optical microscope excitation wavelength needed (Fig. 2a). The reflected light with sample stage on which the AFM sample is mounted needs to be the emission wavelength of the fluorophore (Fig. 1, green arrow) moved. In both cases, there is a loss of AFM performance. In the is filtered again in the fluorescence filter cube (Fig. 1, label 6) to be tip-scanner configuration, the laser detection must be coupled to recorded exclusively and with low background noise on a CCD the moving cantilever, while for the second case, the sample stage camera (Fig. 1, label 7). A large-area visualization camera is used that needs to be moved is complex and heavy. Both types of to align the laser on the tip and place the sample holder over the structures are prone to capture environmental noise and feature tip for easy tip engagement (Fig. 1, yellow arrows). The switching innate resonance frequencies. Furthermore, massive objects do between the laser alignment camera exit located further down in not allow sub-millisecond mechanical response, thus precluding the optical path than the fluorescence or bright field CCD camera individual protein imaging at high resolution and high speed. light recovery pathway is done with an optical switch 45° mirror In this work, we integrate an OM path into our HS-AFM, (Fig. 1, dashed outline) that can be controlled with a linear motor maintaining the structure of the HS-AFM setup1, and hence not stage. Finally, for bright field OM, we inject light (Fig. 1, label 3) compromising HS-AFM performance in terms of speed and from the side into a specially designed novel sample support—a resolution. To achieve this, we choose a completely different cube composed of two aluminum-coated prisms of 1.5-mm edge approach compared with most (if not all) AFM/OM integration length (Fig. 1, label 5; Fig. 2c,d). The bright field light is developments: instead of building a table-top AFM mountable on transmitted through a thin (o100 mm) mica sample support— an inverted optical microscope, accepting loss of AFM like a light table—and is finally recorded on the CCD camera performance, we build an optical path into our HS-AFM setup, (Fig. 1, white arrows). All light is funneled into and out of the accepting minimal trade-offs in OM performance. We show that sample chamber through the same objective1. The physical our setup can acquire bright field and fluorescence OM images of uncoupling of the light sources and the CCD camera (Fig. 1, biological samples, and that it allows HS-AFM tip positioning elements shaded in light blue) from the HS-AFM main body with high precision guided by OM. Finally, we show that it prevents transfer of mechanical destabilizations, such as achieves HS-AFM imaging of individual membrane proteins on vibrational noise, from these elements to the HS-AFM. This eukaryotic cells and record their dynamics at an imaging rate of HS-AFM/OM hybrid has uncompromised HS-AFM imaging 960 ms per frame. This accomplishment opens the door to a wide performance. range of real-time studies of molecular dynamics in membrane processes on living cells. HS-AFM imaging of cells guided by OM. The HS-AFM/OM hybrid setup was operated with short cantilevers (Fig. 3a), Results 6 mm long, 2 mm wide and 100 nm thick, with a high aspect Development of hybrid HS-AFM and fluorescence microscope. ratio tip centred at its end (Fig. 3a, inset). These cantilevers In order to integrate an OM path into the HS-AFM (Fig. 1 and are identical to those used for molecular HS-AFM imaging7. Fig. 2a,b), we added and exchanged several elements to and from In bright field OM, the cantilever appears as a black bar the HS-AFM setup, previously designed by Ando et al1. The (Fig. 3b, left). Two-dimensional cross correlation of the bright original laser diode of the HS-AFM system that reads the field image of the cantilever with a computed black circle of 2 mm cantilever position was changed to a far-red superluminescent diameter (Fig. 3b, inset) results in a cross-correlation map, in diode (SLD) with 750 nm wavelength and low coherence (Fig. 1, which the tip localization is characterized by a cross-correlation label 1). The use of the SLD750 nm allowed the optical separation value maximum (Fig.
Details
-
File Typepdf
-
Upload Time-
-
Content LanguagesEnglish
-
Upload UserAnonymous/Not logged-in
-
File Pages8 Page
-
File Size-