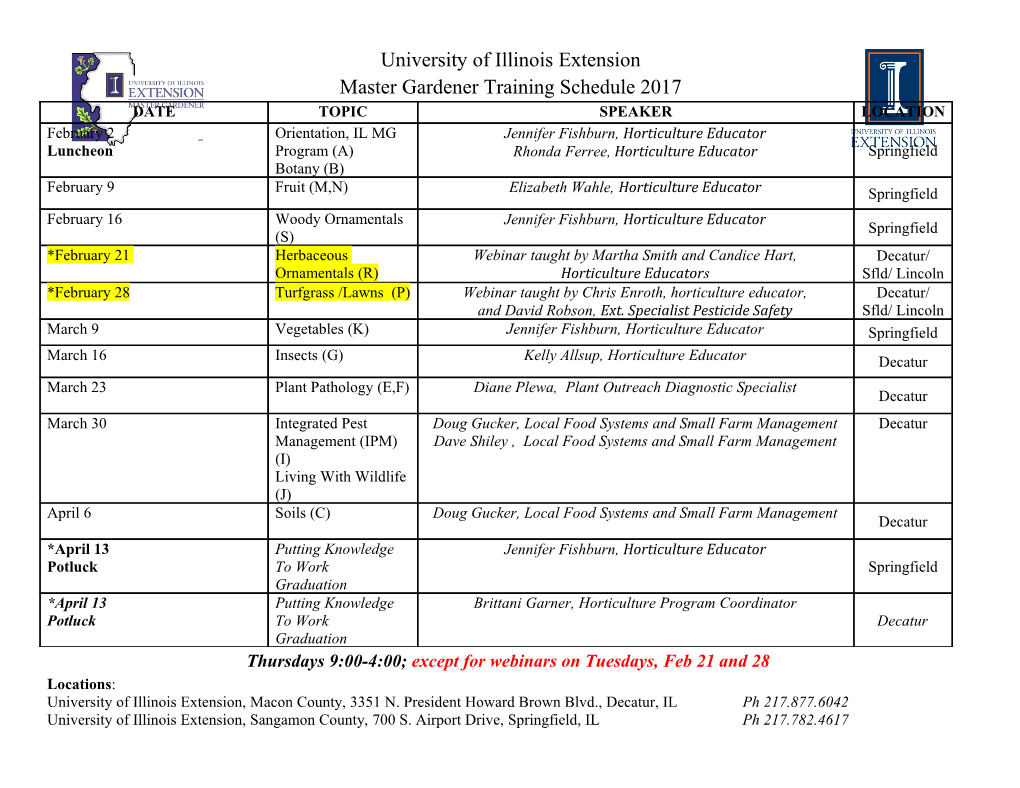
Chapter 10 IONOSPHERICRADIO WAVE PROPAGATION Section 10.1 S. Basu, J. Buchau, F.J. Rich and E.J. Weber Section 10.2 E.C. Field, J.L. Heckscher, P.A. Kossey, and E.A. Lewis Section 10.3 B.S. Dandekar Section 10.4 L.F. McNamara Section 10.5 E.W. Cliver Section 10.6 G.H. Millman Section 10.7 J. Aarons and S. Basu Section 10.8 J.A. Klobuchar Section 10.9 J.A. Klobuchar Section 10.10 S. Basu, M.F. Mendillo The series of reviews presented is an attempt to introduce in HF communications is leading to a rejuvenation of the ionospheric radio wave propagation of interest to system global ionosonde network. users. Although the attempt is made to summarize the field, the individuals writing each section have oriented the work 10.1.1.1 Ionogram. Ionospheric sounders or ionosondes in the direction judged to be most important. are, in principle, HF radars that record the time of flight or We cover areas such as HF and VLF propagation where travel of a transmitted HF signal as a measure of its ionos- the ionosphere is essentially a "black box", that is, a vital pheric reflection height. By sweeping in frequency, typically part of the system. We also cover areas where the ionosphere from 0.5 to 20 MHz, an ionosonde obtains a meas- is essentially a nuisance, such as the scintillations of trans- urement of the ionospheric reflection height as a function ionospheric radio signals. of frequency. A recording of this reflection height meas- Finally, we have included a summary of the main fea- urement as a function of frequency is called an ionogram. tures of the models being used at the time of writing these Ionograms can be used to determine the electron density reviews. [J. Aarons] distribution as a function of height, Ne(h), from a height that is approximately the bottom of the E layer to generally the peak of the F2 layer, except under spread F conditions 10.1 MEASURING TECHNIQUES or under conditions when the underlying ionization prevents measurement of the F2 layer peak density. More directly, ionosondes can be used to determine propagation conditions 10.1.1 Ionosonde on HF communications links. Two typical ionograms produced by a standard analog For more than four decades, sounding the ionosphere ionosphericsounder using film recording techniquesare shown with ionospheric sounders or ionosondes has been the most in Figure 10-1. The frequency range is 0.25 to 20 MHz important technique developed for the investigation of the (horizontal axis), and the displayed height range is 600 km, global structure of the ionosphere, its diurnal, seasonal and with 100km height markers. The bottom ionogram is typical solar cycle changes, and its response to solar disturbances. for daytime, showing the signatures of reflections from the Even the advent of the extremely powerful incoherent scatter E, F1 and F2 layers. The cusps, seen at various frequencies radar technique [Evans, 1975], which permits measurement (where the trace tends to become vertical) indicate the so- of the complete electron density profile, electron and ion called critical frequencies, foE, foF1, and foF2. The critical temperatures, and ionospheric motions, has not made the frequencies are those frequencies at which the ionospheric relatively inexpensive and versatile ionosonde obsolete. On sounder signals penetrate the respective layers. These fre- the contrary, modern techniques of complex ionospheric quencies are a measure of the maximum electron densities parameter measurements and data processing [Bibl and of the respective layers. Since the densities vary with time, Reinisch, 1978a; Wright and Pitteway, 1979; Buchau et al., ionosphericsounding is used to obtain informationon changes 19781 have led to a resurgence of interest in ionospheric in the critical frequency and other parameters of the electron sounding as a basic research tool, while a renewed interest density vs height profile. 10-1 CHAPTER 10 km 700 600 400 NIGHT AM BAND 300 .25 2 3 4 5 6 7 8 9 10 5 20 MHz DAY Figure 10-1. Typical midlatitude day and nighttime ionograms, recorded by a C-4 ionosonde at Boulder, Colorado. The daytime ionogram shows reflections from E, Es, F1 and F2 layers; the nighttime ionogram those from Es and F2 layers. The ionogram (Figure 10-1) shows signatures of various Finally, we see vertical bands in the frequency range phenomena that complicate the process of ionospheric from 0.5 to 1.7 MHz, the signature of radio frequency sounding or the ionogram analysis. Superimposed on the interference (RFI) in an ionogram, here from the AM band. primary F layer echo trace is a similar but not identical RFI can become severe enough to prevent the recording of trace, shifted up in frequency: the so-called extraordinary ionospheric echoes; for example, interference masks part or or X component. The primary trace is called the ordinary all of the E layer trace below 1.7 MHz. or 0 component. The echo trace is split into two traces due The top of Figure 10-1 shows a typical nighttime ion- to effects of the earth's magnetic field. A second trace sim- ogram. The E and F1 traces have disappeared because these ilar to the primary trace is seen at twice the range, a multiple layers dissipate after sunset. (Residual nighttime E region reflection. Only a small fraction of the wave energy is re- ionization of low density can be observed in the absenceof ceived by the antenna after it has returned from the iono- low sporadic E layers at stations with low RF1 and large sphere. Most of the returned energy is reflected back from antennas.) Echoes from a sporadic E layer (Es) and F2 layer the ground and provides the first multiple (second order echoes and their multiples are clearly visible in Figure echo) at twice the range. If the ionosphere is a good reflector, 10-1. At times a brushlike spreading of the F2 layer cusps and losses in the D region are low, additional reflections is observed. It is called spread F and is caused by small can be observed. Figure 10-1 (Night) shows a second mul- scale irregularities embedded in the ionosphere and ripples tiple (third order echo) for part of the Es trace. It is easy in the equidensity contours on the order of hundreds of to see that slopes increase by a factor that corresponds to meters to kilometers. For a detailed discussion of spread F the order of the echo. see Davies [1966] and Rawer and Suchy [19671; for a dis- 10-2 IONOSPHERIC RADIO WAVE PROPAGATION cussion of the occurrence and global distribution see Herman f = 0.009 N (10.4) [19661. The nighttime ionogram also shows increased RFI bands at higher frequencies. Because the D layer disappears Ne = 1.24 x 104 f2n (10.5) at night, HF propagation over large distances is possible. This long distance propagation is heavily used for broad- where fN is in MHz and Ne in electrons/cm3 . The plasma casting by commercial users and for shortwave radio com- frequency is the natural frequency of oscillation for a slab munications by government services and radio amateurs. of neutral plasma with the density Ne after the electrons Fortunately the ionosonde's own echoes also increase in have been displaced from the ions and are allowed to move amplitude due to the disappearance of the D layer, reducing freely. For further discussions of the relation of u to the to some extent the effect of increased propagated noise on wave propagation see Davies [1966]. the systems overall signal-to-noise ratio. Peak densities of the ionospheric layers vary between l0 4 and > 106el/cm 3 . Inserting these numbers into Equation 10.1.1.2 Principles of Ionospheric Sounding. The con- (10.4) gives a plasma frequency range from 1 to > 9 MHz; cept of ionospheric sounding was born as early as 1924, this is the reason for the frequency range (0.5 MHz <f < when Breit and Tuve [1926] proved the existence of an 20 MHz) covered by a typical ionosonde. The low densities ionized layer with the reception of ionospheric echoes of of the D layer can only be probed with low frequencies HF pulses transmitted at 4.3 MHz from a remote transmitter < 250 Hz, requiring large antennas and complex processing/ (distance 13.8 km). This, during the next decade, led to the analysis techniques and are not directly measurable by the development of monostatic ionospheric sounders by the Na- standard ionosondes (for details see Kelso [1964] and ref- tional Bureau of Standards and the Carnegie Institution. erences therein). Indirectly the D region ionization is meas- Even today the principles used by Breit and Tuves constitute ured by the integral absorption effects that it imposes on the principles on which most ionospheric sounders are based. the HF waves propagating through it to the E or F region These are the transmission of HF pulses and the measure- reflection levels (see discussion of fmin). ment of their time of flight to the reflection level. For a The inclusion of the magnetic field in the formula for short historical review of the development of ionospheric the refractive index leads to the well known Appleton dis- sounders see Villard [1976]. persion formula (dispersion means that the refractive index Ionospheric sounding takes advantage of the refractive depends on the propagating frequency) for a magnetized properties of the ionosphere. A radio wave propagating into plasma, here given for the case of no collisions, generally the ionospheric plasma encounters a medium with the re- valid for frequencies > 2 MHz, in the E and F regions.
Details
-
File Typepdf
-
Upload Time-
-
Content LanguagesEnglish
-
Upload UserAnonymous/Not logged-in
-
File Pages111 Page
-
File Size-