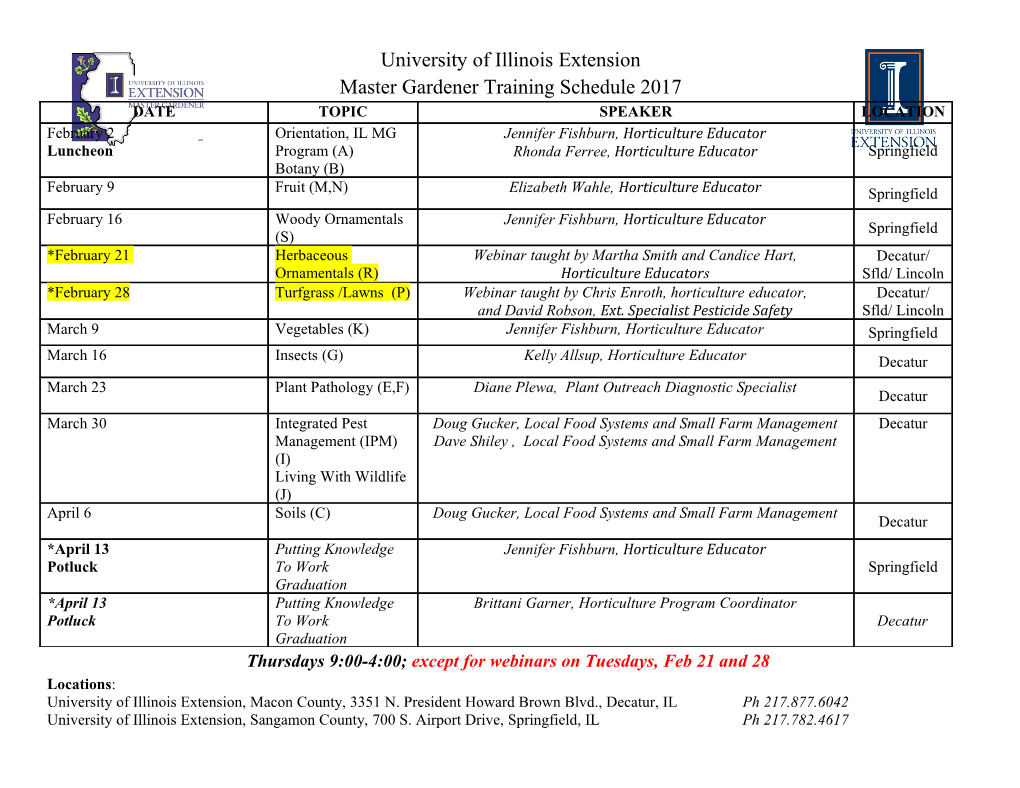
PUBLICATIONS Journal of Geophysical Research: Planets RESEARCH ARTICLE Electromagnetic signal penetration in a planetary soil 10.1002/2016JE005192 simulant: Estimated attenuation rates using GPR Key Points: and TDR in volcanic deposits on Mount Etna • GPR methodologies for evaluating the loss tangent of volcanic sediments S. E. Lauro1 , E. Mattei1 , B. Cosciotti1 , F. Di Paolo1 , S. A. Arcone2, M. Viccaro3,4 , • Characterization of electrical 1 properties of a planetary soil simulant and E. Pettinelli • Comparison between GPR and TDR 1 2 measurements Dipartimento di Matematica e Fisica, Università degli Studi Roma TRE, Rome, Italy, US Army ERDC-CRREL, Hanover, New Hampshire, USA, 3Dipartimento di Scienze Biologiche Geologiche e Ambientali, Università degli Studi di Catania, Catania, Italy, 4Osservatorio Etneo, Istituto Nazionale di Geofisica e Vulcanologia, Catania, Italy Correspondence to: S. E. Lauro, Abstract Ground-penetrating radar (GPR) is a well-established geophysical terrestrial exploration method [email protected] and has recently become one of the most promising for planetary subsurface exploration. Several future landing vehicles like EXOMARS, 2020 NASA ROVER, and Chang’e-4, to mention a few, will host GPR. A GPR Citation: survey has been conducted on volcanic deposits on Mount Etna (Italy), considered a good analogue for Lauro, S. E., E. Mattei, B. Cosciotti, F. Di Paolo, S. A. Arcone, M. Viccaro, and Martian and Lunar volcanic terrains, to test a novel methodology for subsoil dielectric properties estimation. E. Pettinelli (2017), Electromagnetic The stratigraphy of the volcanic deposits was investigated using 500 MHz and 1 GHz antennas in two different signal penetration in a planetary soil configurations: transverse electric and transverse magnetic. Sloping discontinuities have been used to simulant: Estimated attenuation rates using GPR and TDR in volcanic deposits estimate the loss tangents of the upper layer of such deposits by applying the amplitude-decay and on Mount Etna, J. Geophys. Res. Planets, frequency shift methods and approximating the GPR transmitted signal by Gaussian and Ricker wavelets. The 122, 1392–1404, doi:10.1002/ loss tangent values, estimated using these two methodologies, were compared and validated with those 2016JE005192. retrieved from time domain reflectometry measurements acquired along the radar profiles. The results show Received 7 OCT 2016 that the proposed analysis, together with typical GPR methods for the estimation of the real part of Accepted 11 MAY 2017 permittivity, can be successfully used to characterize the electrical properties of planetary subsurface and to Accepted article online 15 MAY 2017 define some constraints on its lithology of the subsurface. Published online 3 JUL 2017 1. Introduction Ground Penetrating Radar (GPR) has been extensively applied to a large number of terrestrial applications such as glaciology, engineering, archaeology, geology, and environmental and agricultural sciences [Jol, 2008, and references therein]. GPR uses radio waves to image the buried geological structures in a way conceptually similar to seismic reflection methods. The application of GPR to planetary exploration is particu- larly favorable for two main reasons: (1) planetary subsurfaces are generally dry and cold, which minimizes the attenuation and dispersion of the electromagnetic waves; and (2) radar measurements do not require a physical contact between antenna and soil. As a consequence, GPR can be designed to operate either remotely, on board a spacecraft, or on site as part of a payload of a landing vehicle (rover or lander). These different operating modes were first tried during the pioneering electromagnetic experimentation conducted during the Apollo 17 mission: the Surface Electrical Properties (SEP) experiment was hosted on board the Lunar Roving Vehicle [Simmons et al., 1973] and the Apollo Lunar Sounder Experiment (ALSE) on board the Apollo Command and Service Module [Porcello et al., 1974]. In the last 20 years, several orbiting GPR have been proposed, designed, and built (RIME [Bruzzone et al., 2013] and REASON [Phillips and Pappalardo, 2014]) as well as successfully used in various missions (MARSIS [Plaut et al., 2007], SHARAD [Phillips et al., 2008], Kaguya Lunar Radar Sounder (LRS) [Ono et al., 2010], CONSERT [Kofman et al., 2015], and Chang’E-3 Lunar Penetrating Radar (LPR) [Fang et al., 2014]). Furthermore, several landing missions to Mars and the Moon will rely on GPR to investigate geological structures at shallow depth (RIMFAX [Hamran et al., 2015] and China’s Mars 2020 mission [Zhou et al., 2016]) and/or to guide drilling for subsurface soil sampling (WISDOM [Dorizon et al., 2016]). The Chinese lunar mission CHANG’E-3 has already attempted dual-frequency GPR [Fang et al., 2014]. Radar data can provide qualitative and quantitative information that can be integrated to reconstruct the geological struc- fi ©2017. American Geophysical Union. ture of the subsurface. Quantitative information is more dif cult to retrieve because it requires the imple- All Rights Reserved. mentation of inverse algorithms [e.g., Zhang et al., 2008; Lauro et al., 2017]. Nevertheless, the extraction of LAURO ET AL. EM SIGNAL PENETRATION IN PLANETARY SOIL 1392 Journal of Geophysical Research: Planets 10.1002/2016JE005192 the complete set of dielectric parameters, that is, the real part of permittivity, the polarization term, and the conductivity strongly improves the interpretation of the composition of the planetary subsurface. The potentials of this approach can be seen in the detailed reconstruction of the Moon shallow subsurface structure, which is well constrained by the variation of permittivity and loss tangent with depth [Strangway et al., 1977]. The present work is dedicated to improve our capability to retrieve quantitative information on the electrical nature of a planetary subsurface and discuss some of the problems encountered in data analysis and inter- pretation when using a high-frequency GPR in proximity of the planetary surface [Vannaroni et al., 2004; Fang et al., 2014], that is, when the radar is on board a rover or a lander. As is well known, GPR data are not self-consistent. For example, in a radar cross section the positions of different subsurface interfaces (or of the single scatterers) are ranged in time delay (specifically two-way travel time) and aspect (look angle), and electromagnetic wave velocity is needed to convert time into depth. Therefore, detailed and accurate data interpretation requires additional information regarding the media velocity and attenuation (which depend on the real and imaginary parts of permittivity), especially if the final goal is the identification of the subsurface lithology, as any single velocity or attenuation value can be associated with more than one type of rock (or soil). On the Earth the electromagnetic parameters can be independently measured on site using complementary techniques (e.g., time or frequency domain techniques), whereas in a planetary mission some ad hoc pro- cedure must be developed. Several methods have been proposed to estimate the electromagnetic pulse velocity: (i) hyperbola calibration [Annan, 2004]; (ii) surface reflectivity (in case of noncontact antennas) [e.g., Lambot et al., 2004]; (iii) early-time attribute (for ground coupled antennas) [Pettinelli et al., 2014]; and (iv) multioffset antenna configuration [Jol, 2008; Annan, 2004; Huisman et al., 2003]. However, among these, only the hyperbola calibration method appears suitable for planetary exploration because it can be applied to a single offset antenna configuration (fixed position of the Tx-Rx antennas) and does not require any cali- bration. On the other hand, in GPR studies, the attenuation factor is commonly evaluated using spectral ratio [Harbi and McMechan, 2012], frequency shift [Liu et al., 1998; Bradford, 1999], or amplitude-decay [Tonn, 1991; Grimm et al., 2006]; in principle, almost all seismic reflection methods [Tonn, 1991] could be implemented for GPR. The only drawback associated with such techniques is the need for a buried sloping horizon. In this work we focus on estimating the loss tangent (attenuation) from GPR data acquired on volcanic depos- its we consider good petrological analogues of various terrains present in all terrestrial planets [Wilson and Head, 1994; Pettinelli et al., 2003] and even on comets [Brouet et al., 2014]. The survey was performed using a commercial GPR equipped with soil coupling antennas. The work is strictly methodological, as the survey could have been performed at any other site of interest for planetary exploration and the proposed analysis can be extended to other types of radar systems equipped with suspended (uncoupled) antennas. The data were collected on two different volcanic deposits which were fairly homogeneous at the scale of the radar wavelength. Under the assumption that such deposits were low loss and weakly dispersive, we estimated the loss tangent by applying two different methods, the amplitude decay and the frequency shift in the portion of radar cross sections where dipping reflectors were well detectable. The results were validated using time domain reflectometry (TDR) measurements collected at various depths along the GPR profiles and compared with radar data collected on the Moon and Mars to highlight the potentials of the proposed approach. 2. Theoretical Background 2.1. Wave Propagation Parameters The propagation of GPR signals depends on the constitutive parameters
Details
-
File Typepdf
-
Upload Time-
-
Content LanguagesEnglish
-
Upload UserAnonymous/Not logged-in
-
File Pages13 Page
-
File Size-