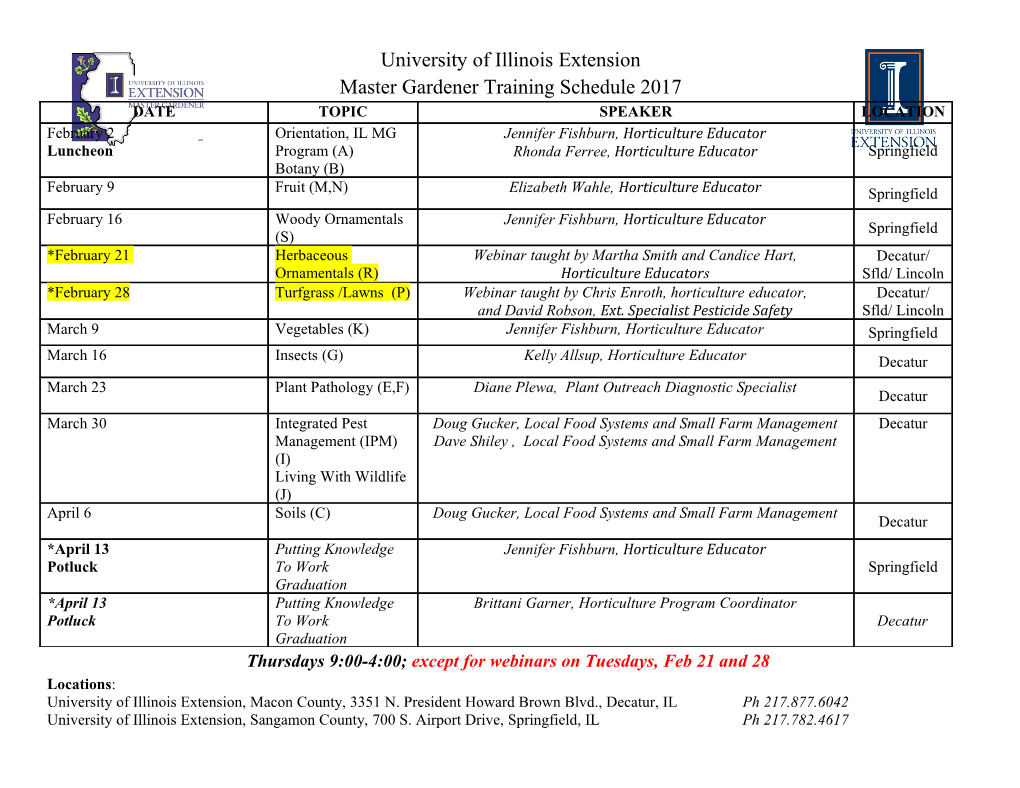
Quantum Chemistry A Concise Reference of Formulae, Concepts & Data Dr. Wissam Helal, Department of Chemistry, The University of Jordan, [email protected] October 10, 2020 About this document This document is intended to be a concise desk reference of quantum chemistry to be used by both undergraduate and graduate students. It contains three main parts: basic formulas, concepts, and symbols of quantum chemistry (sections 1 – 6); a short review of relevant mathematics and classical physics (sections 7 & 8); and some miscellaneous other useful data (sections 9 & 10). It should be emphasized that this document is not intended to be a summary of any quantum chemistry course, but rather, a useful handout to be used when working out problems. Moreover, this short refernce is far to be comprehensive and do not cover all aspects of the field. Contents 7 Mathematics 11 7.1 Algebra, Geometry & Trigonometry ...... 11 1 Quantum Mechanics 2 7.2 Derivatives & Integrals .............. 12 1.1 Formulae of Quantum Mechanics ........ 2 7.3 Table of Integrals ................. 12 1.2 Postulates of Quantum Mechanics ....... 3 7.4 Power Series .................... 13 1.3 Dirac Notation (Bracket Notation) ....... 3 7.5 Spherical Polar Coordinates ........... 13 1.4 Theorems of Quantum Mechanics ........ 3 7.6 Complex Numbers ................ 13 7.7 Vectors ...................... 14 2 Systems with Exact Solutions 4 7.8 Determinants ................... 15 2.1 The Particle in a Box .............. 4 7.9 Simultaneous Linear Equations ......... 15 2.2 The Particle in a Ring .............. 4 7.10 Matrices ...................... 15 2.3 The Harmonic Oscillator ............. 4 7.11 Eigenvalues and Eigenvectors .......... 16 2.4 Angular Momentum ............... 5 2.5 The Rigid Rotor ................. 5 8 Classical Physics 17 2.6 The Hydrogen (Hydrogenlike) Atom ...... 5 8.1 Classical Mechanics ................ 17 8.2 The Classical Wave Equation .......... 18 3 Approximation Methods 7 8.3 Electrostatics ................... 18 3.1 The Variational Method ............. 7 8.4 Magnetism .................... 18 3.2 Perturbation Theory ............... 7 9 Molecular&Spectroscopic Data 19 4 Electron Spin 7 10 Other Useful Data 20 5 Polylectronic Atoms 7 10.1 SI Units & Unit Prefixes ............. 20 5.1 Hamiltonian & Wavefunctions .......... 7 10.2 Energy Conversion Factors ........... 20 5.2 Angular Momenta ................ 8 10.3 Symbols for Elementary Particles ........ 20 5.3 Atomic Term Symbols & Spectra ........ 8 10.4 Fundamental Physical Constants ........ 21 10.5 Atomic Units ................... 21 6 Molecular Electronic Structure 9 10.6 The Greek Alphabet ............... 21 6.1 Molecular Orbital Theory ............ 9 10.7 Periodic Table of the Elements ......... 22 6.2 Molecular Term Symbols ............. 9 10.8 Atomic Masses for Selected Isotopes ...... 22 6.3 H¨uckel MO Theory ................ 9 10.9 Effective Nuclear Charge (Zeff = Z σ) .... 22 6.4 Molecular Symmetry ............... 10 − 6.5 The Born-Oppenheimer Approximation .... 10 1 1 Quantum Mechanics Orthonormalization of functions and Kronecker delta: 1.1 Formulae of Quantum Mechanics 0 for i = j ψ∗ψ dτ = δ , δ = (13) i j ij ij 1 for i =6 j Speed of electromagnetic waves in terms of frequency ν Z and wavelength λ: c = νλ (1) The sum and the difference of two operators Aˆ and Bˆ: Frequency ν and wavelength λ with relation to wavenum- (Aˆ Bˆ)f(x)= Afˆ (x) Bfˆ (x) (14) bersν ˜: 1 ν ± ± ν˜ = = (2) λ c The product of two operators Aˆ and Bˆ: Planck quantization of energy: ˆ ˆ ˆ ˆ E = hν (3) ABf(x)= A[Bf(x)] (15) de Broglie wavelength λ in terms of the particle’s momen- Linear operators should satisfy: Identities of commuta- tum p: h h tors (where k is a constant and the operators are assumed λ = = p mv to be linear): Time-dependent Schr¨odinger equation for one-particle [A,ˆ Bˆ]= [B,ˆ Aˆ] (16) (with mass m), one-dimensional system, in terms of wave- − [A,ˆ Aˆn] = 0, n = 1, 2, 3,... (17) function Ψ and potential energy function V (x,t)(~ = h ): 2π ˆ ˆ ˆ ˆ ˆ ˆ ~ ∂Ψ(x,t) ~2 ∂2Ψ(x,t) [kA, B]=[A,kB]= k[A, B] (18) = + V (x,t)Ψ(x,t) (4) − i ∂t −2m ∂x2 [Aˆ + B,ˆ Cˆ]=[A,ˆ Cˆ]+[B,ˆ Cˆ] (19) The probability at time t of finding the particle in the [A,ˆ Bˆ + Cˆ]=[A,ˆ Bˆ]+[A,ˆ Cˆ] (20) region of the x-axis lying between x and x + dx, for a ˆ ˆ ˆ ˆ ˆ ˆ ˆ ˆ ˆ one-particle, one-dimensional system: [AB, C]=[A, C]B + A[B, C] (21) Ψ(x,t) 2 dx (5) [A,ˆ BˆCˆ]=[A,ˆ Bˆ]Cˆ + Bˆ[A,ˆ Cˆ] (22) | | The function Ψ(x,t) 2 is the probability density, de- Average value of an observable A: fined as | | Ψ 2 =Ψ∗Ψ (6) | | ∗ ∗ A = ψ Aψˆ dτ (23) with Ψ being the complex conjugate of the function Ψ, h i the complex conjugate being formed by replacing i with Z i wherever it occurs. If A and B are any two properties and k is a constant: − A + B = A + B and kA = k A (24) Time-dependent wave function Ψ is a function of time- h i h i h i h i h i independent wavefunction ψ(x) and time function f(t): ~ Ψ(x,t)= ψ(x) f(t)= ψ(x) e−iEt/ (7) However, the average value of a product need not equal the product of the average values: Time-independent Schr¨odinger equation in terms of the ˆ AB = A B (25) Hamiltonian operator H, the wavefunction ψ (or h i 6 h ih i eigenfunction), and energy E (or eigenvalue): pˆ2 Hermitian operators are defined as: Hψˆ = Eψ, Hˆ = Tˆ + V,ˆ Tˆ = q (8) 2m ∗ ˆ ˆ ∗ ψmAψndτ = ψn(Aψm) dτ (26) Linear momentum quantum mech. operator in the q axis: Z Z ~ ∂ pˆq = (9) The variance σ2 and the standard deviation σ (or ∆) i ∂q of an observable A: One-particle three-dimensional Hamiltonian operator: 1/2 ~2 ∂2 ∂2 ∂2 2 2 2 2 2 ˆ 2 2 σA = A A and σA ∆A = A A H = +V (x,y,z), = 2 + 2 + 2 (10) h i−h i ≡ h i−h i −2m∇ ∇ ∂x ∂y ∂z The probability of finding a particle between x1 and x2: Robertson inequality x2 ∗ Probability (x1 x x2)= ψ (x)ψ(x) dx (11) 1 ∗ ˆ ˆ ≤ ≤ σAσB ∆A∆B Ψ [A, B]Ψ dτ Zx1 ≡ ≥ 2 Z The normalization condition in three-dimension: +∞ +∞ +∞ Heisenberg uncertainty relations: position x and linear ∗ ψ (x,y,z)ψ(x,y,z) dxdy dz momentum px, energy E and time t, and angle φ and an- Z−∞ Z−∞ Z−∞ gular momentum L complementaries: = ψ∗ψdτ = 1 (12) ~ ~ ~ ∆x∆p , ∆E∆t , ∆φ∆L (27) Z x ≥ 2 ≥ 2 z ≥ 2 2 ∗ ˆ 1.2 Postulates of Quantum Mechanics The definite integral Ψ1AΨ2dτ is abreviated: Postulate 1 (Wavefunctions): The state of a quantum Ψ Aˆ Ψ 1 Aˆ 2 Ψ∗AˆΨ dτ A (31) h 1| | 2i≡h |R | i≡ 1 2 ≡ 12 mechanical system is completely specified by a state func- An operator Aˆ is said to be HermitianZ if it satisfies: tion, Ψ(r, t), that is a function of the coordinates of the ψ Aˆ ψ = ψ Aˆ ψ ∗ ψ∗ Aψˆ dτ = ψ (Aψˆ )∗dτ particles r and the time t. If time is not a variable, its h m| | ni h n| | mi ≡ m n n m Z Z state is completely specified by a time-independent wave- 1.4 Theorems of Quantum Mechanics function ψ(r). All possible information about the system Hermitian operators: can be derived from Ψ(r, t). These wavefunctions are well behaved: single-valued, continuous, and quadratically in- 1. The eigenvalues of a Hermitian operator are real. ˆ tegrable. 2. Two eigenfunctions of a Hermitian operator A that correspond to different eigenvalues are orthogonal. Postulate 2 (Operators): To every observable in classical Eigenfunctions of Aˆ that belong to a degenerate mechanics there corresponds a linear Hermitian quantum eigenvalue can always be chosen to be orthogonal. mechanical operator. The operator is obtained from the 3. Let the functions g1 , g2 , ... be the complete set of classical mechanical expression for the observable written eigenfunctions of the Hermitian operator Aˆ, and let in terms of Cartesian coordinates and corresponding lin- the function Ψ be an eigenfunction of Aˆ with eigen- ear momentum components by replacing each coordinate value k. Then if Ψ is expanded as Ψ = aigi q by itself and the corresponding momentum component i ≡ Ψ = i gi gi Ψ , the only nonzero coefficients ai pq by i~ ∂/∂q. | i | ih | i P − are those for which gi has the eigenvalue k. Postulate 3 (Eigenvalues): In any measurement of the These threeP theorems can be summarized as: the eigen- physical observable A associated with the operator Aˆ, the functions of a Hermitian operator form a complete, or- only values that will ever be observed are the eigenvalues thonormal set, and the eigenvalues are real. ai, which satisfy the eigenvalue equation Commuting operators: AˆΨi = aiΨi 4. If the linear operators Aˆ and Bˆ have a common com- ˆ ˆ The eigenfunctions Ψi are required to be well-behaved. plete set of eigenfunctions, then A and B commute. Postulate 4 (Completeness): If Aˆ is a linear Hermitian 5. If [A,ˆ Bˆ]=0(Aˆ and Bˆ Hermitian), we can select a operator that represents a physically observable property, common complete set of eigenfunctions for them. then the eigenfunctions gi of Aˆ form a complete set. 6. If gm and gn are eigenfunctions of the Hermitian operator Aˆ with different eigenvalues (that is, if Postulate 5 (Average values): If Ψ(r, t) is the normal- Agˆ = a g and Agˆ = a g with a = a ), ized state function of a system at time t, then the average m m m n n n m n and if the linear operator Bˆ commutes with A6ˆ, then value of the observable A corresponding to Aˆ is g Bˆ g = 0 for a = a .
Details
-
File Typepdf
-
Upload Time-
-
Content LanguagesEnglish
-
Upload UserAnonymous/Not logged-in
-
File Pages22 Page
-
File Size-