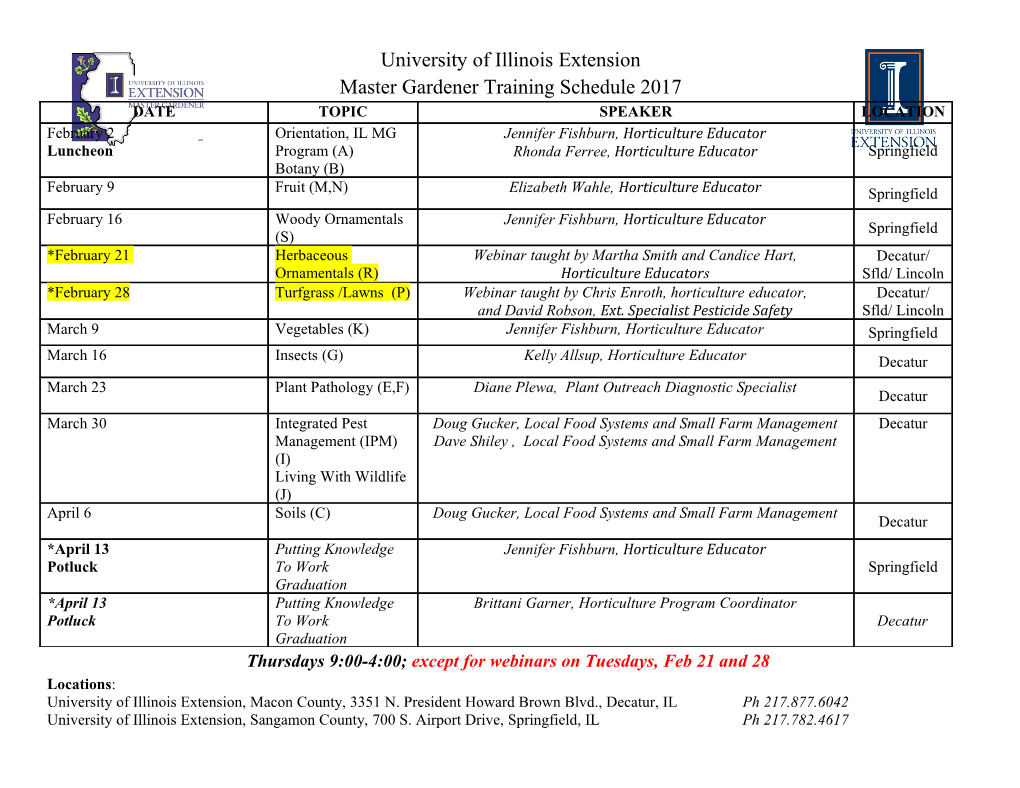
Journal of Structural Geology 118 (2019) 68–86 Contents lists available at ScienceDirect Journal of Structural Geology journal homepage: www.elsevier.com/locate/jsg Integrating kinematic restoration and forward finite element simulations to constrain the evolution of salt diapirism and overburden deformation in T evaporite basins ∗ J. Ryan Thigpena, , Dan Robertsb, J. Kent Snowc, Christopher D. Walkerc, Adam Bereb a Department of Earth and Environmental Sciences, University of Kentucky, Lexington, KY, 40506, USA b Rockfield Global, Ethos, Kings Road, Prince of Wales Dock, Swansea Waterfront, SA1 8AS, UK c BP America, 501 Westlake Park Boulevard, Houston, TX, 77079, USA ABSTRACT In evaporite basins, salt deformation including inflation, diapirism, and salt canopy emplacement is inherently non-coaxial and ductile and thus it presents challenges for two-dimensional kinematic restorations that rely on line-length and area-balancing assumptions. Also, because salt flow and the resulting deformation of adjacent cover units can be driven by temporally and spatially transient salt pressure gradients, kinematic restorations are generally unable to predict the magnitude and distribution of subseismic deformation that results from a particular structural scenario. Here, we use a case study from the Atwater fold belt, Gulf of Mexico, to test a new workflow that involves comparison of kinematic restoration models with forward numerical (finite-element) models of structural evolution to examine the physical validity of solutions derived from the kinematic restorations and to determine the nature and spatial distribution of the resultant subseismic deformation. In the Atwater fold belt, which represents the downdip portion of a linked updip (landward) extensional-downdip (seaward) contractional system, our kinematic restorations indicated that major anticlines likely result from early short wavelength folding followed by (1) inflation of the autochthonous salt to drive failure of the overburden, (2) collapse of the updip limb of the major salt-cored anticline as the salt evacuates updip, and (3) rapid emplacement of the allochthonous salt canopy. In margin scale finite element models of the same system, progradation of the sedimentary wedge above the weak salt substrate leads to basinward migration of the salt and produces inflation of the major downdip salt-cored folds, as predicted by the kinematic model. However, in relatively strong overburden materials (equivalent friction angle = 32°), such salt flow only sustains inflation of the anticlines and is unable to reproduce the interpreted collapse of the anticlinal backlimb or emplacement of the salt canopy. Alternate model runs that include a significant reduction in material strength (equivalent friction angle = 18°) allow salt in the anticlinal crest to drive both reactive and active diapirism and ultimately lead to rapid emplacement of allochthonous canopies. In all of these models, diapirism drives substantial seismically-resolvable and subseismic deformation of wall rocks. Additionally, these models clearly show that the stress field, and particularly the K value (horizontal-vertical stress ratio) of the sediments adjacent to salt structures used for estimating stress magnitudes for drilling predictions, is fundamentally dependent on what point along the evolutionary path from autochthonous salt, to diapir, to salt sheet, that each structure resides. These results highlight the need to test complex kinematic restorations with physics-based techniques. Additionally, they demonstrate that integrating kinematic restorations with these finite element solutions can substantially increase our ability to predict both subseismic reservoir damage in sediments adjacent to salt structures and the K values used for forecasting drilling conditions, particularly in young basins filled with poorly consolidated sediments. 1. Introduction basin, the genesis of the major deepwater fields is directly tied to salt flow, including diapir evolution. The evolution of these features exerts Many of the world's prolific hydrocarbon provinces lie in passive tremendous influence on the structural geometry of overburden units margin salt basins (e.g., Gulf of Mexico, Persian Gulf, North Sea, Lower and the consequent compartmentalization of prospects, the deposi- Congo, Campos, and Precaspian basins; Hudec and Jackson, 2007; tional paleobathymetry, and the resultant distribution of lithofacies, Rowan and Ratliff, 2012) and many major deepwater fields in these which directly drives field developmental planning (i.e., location and basins are influenced by salt structures. Along these evaporite-floored number of wells). In these geologically young systems that include passive margins, gravity driven collapse and basin prograding sedi- poorly consolidated sediments, salt pressure can drive plastic de- mentation leads to development of an updip extensional zone that is formation that can be both pervasive (accelerated compaction; Weller, kinematically linked with a downdip contractional system along a 1959) and discrete (deformation bands; Schultz and Siddharthan, 2005) common master detachment (Fig. 1; Wu et al., 1990; Demercian et al., and lead to unpredicted reservoir flow “baffling”. Additionally, because − 1993; Letouzey et al., 1995; Peel et al., 1995). In the Gulf of Mexico viscous salt flow rates can result in strain rates much higher (10 8 to ∗ Corresponding author. E-mail address: [email protected] (J.R. Thigpen). https://doi.org/10.1016/j.jsg.2018.10.003 Received 2 April 2018; Received in revised form 3 October 2018; Accepted 3 October 2018 Available online 06 October 2018 0191-8141/ © 2018 Elsevier Ltd. All rights reserved. J.R. Thigpen et al. Journal of Structural Geology 118 (2019) 68–86 Fig. 1. (a) Bathymetric map of the northern Gulf of Mexico and southernmost onshore Louisiana. Position of section line from Fig. 1b is shown. The Atwater fold belt lies directly beneath the toe of the allochthonous Sigsbee salt canopy, which is clearly expressed in the present-day bathymetry. NO – New Orleans. MC – Mississippi Canyon. AT – Atwater Valley. GC – Green Canyon. WR – Walker Ridge. GB – Garden Banks. KC – Keathley Canyon. Modified from Pilcher et al. (2011). (b) Schematic diagram of a gravity-driven, linked updip extensional and downdip contractional system separated by a transition zone. The updip extensional normal faulting and downdip contractional folding and thrust faulting are kinematically linked along the weak basal salt detachment. Approximate position of section line shown in (a). Modified from Applied Geodynamics Laboratory, UT-Austin. (For interpretation of the references to colour in this figure legend, the reader is referred to the Web version of this article.) − − 10 12 s 1; Jackson and Talbot, 1986) than those observed in most mechanisms of major salt structures and their influence on the temporal − − − geologic systems (10 13 to 10 15 s 1; Pfiffner and Ramsay, 1982), salt and spatial evolution of the stress field, with a particular emphasis on flow can impact rate-dependent processes such as salt-sediment loading how such stress evolution may impact drilling predictions. Although rates, sediment dewatering, and advectionary heat transport, thus al- hydrocarbon fields can form throughout these evaporite systems, this lowing salt tectonics to substantially impact pore pressure, basin case study focuses exclusively on the evolution of major anticlinal traps thermal evolution, and maturation processes. Despite this, many first- cored by salt, similar to many downdip structures in passive margin order components of salt evolution remain poorly understood, and as Atlantic basins. result, evolutionary models for many of these fields may vary. Kinematic restorations are fundamental tools for testing structural interpretations in both industry and academia. However, they are often 2. Passive margin salt basins based purely on geometric line length and area balancing assumptions and rarely yield unique solutions, especially in settings that involve Although passive margin salt basins display a broad range of non-coaxial deformation and volumetric strain such as evaporite basins. structural styles, most of them include an updip (landward) extensional Additionally, as finite deformation (and hence structural damage) is zone dominated by listric normal faulting, rollover expulsion, and re- dependent not only on the final structural geometry but also the stress active diapirism, a mid-slope transition/translational zone, and a down- and strain paths (Karig and Hou, 1992), comprehensive characteriza- dip (seaward) contractional zone dominated by salt-cored folds, thrust tion of strain damage requires an understanding of the forces driving faults, active diaprism, and extrusive salt sheets and canopies (Hudec deformation. Ideally, restorations would be carried out while keeping a and Jackson, 2007, Fig. 1). These zones are kinematically linked fl strain “budget”, yet constitutive equations governing deformation me- through evaporite detachment horizons, where salt layers ow beneath chanics cannot be run in reverse thus, it is impossible to carry out relatively-thick overburden strata (up to 10 km thick), leading to dis- crete and pervasive extensional and contractional overburden de- numerical tasks such as “uncreeping” salt. However, it may be possible to evaluate the viability of structural evolution models by creating formation, diapirism, and allochthonous canopy formation. At the margin scale, salt flow is dominantly driven by gravitational
Details
-
File Typepdf
-
Upload Time-
-
Content LanguagesEnglish
-
Upload UserAnonymous/Not logged-in
-
File Pages19 Page
-
File Size-