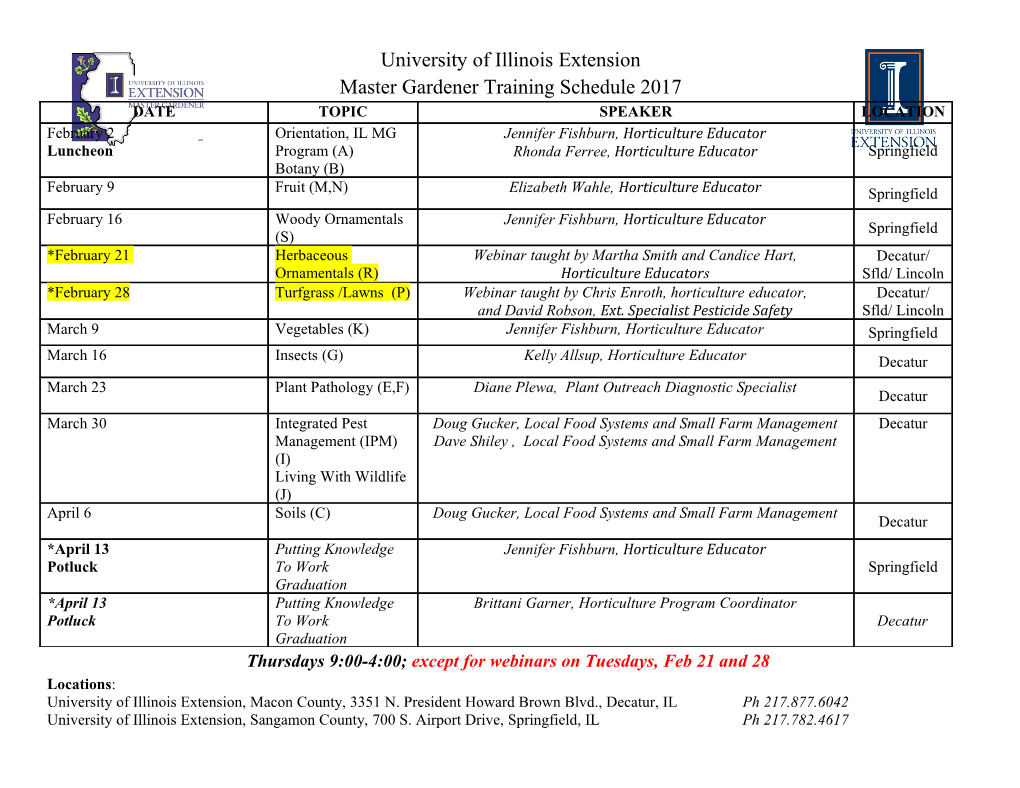
Journal of the American Mosquito Control Association, 12(2):372_379, 1996 Copyright O 1996 by the American Mosquito Control Association, Inc. INFLUENCE OF AIRCRAFT VORTICESON SPRAY CLOUD BEHAVIOR R. E. MICKLE Atmospheric Environment Service, 4905 Dufferin Street, Downsview, Ontario M3H5T4, Canada ABSTRACT. For small droplet spraying, the spray cloud is initially entrained into the wingtip vortices so that the ultimate fate of the spray is conffolled by the motion of these vortices. In close to 10O aerial sprays, the emitted spray cloud has been mapped using a scanning laser system that displays diffusion and transport of the spray cloud. Results detailing the concentrations within the spray cloud in space and time are given for sprays in parallel and crosswinds. Wind direction is seen to potentially alter the vortex motion and hence the fate of the spray cloud. In crosswind spraying, the vortex behavior associated with the 2 wings is found to differ, which leads to enhanced deposition from the upwind wing and enhanced drift from the downwind wins. INTRODUCTION EXPERIMENTAL METHODS The application of larvicides and adulticides The ARAL (AES Rapid Acquisition Lidar) by aircraft has been an effective mechanism for has been used in 2 experiments to map close to mosquito control. Howevet the benefits of 100 different spray scenarios in a variety of me- spraying are accompanied by the difficulty in teorological conditions (stable and unstable) en- targeting small droplets and by the potential en- compassing light to high winds at cross and par- vironmental impact of applying any chemical allel angles to the flight line. Aircraft used in into the environment in quantities that may be these studies have included a Cessna 188, TBM toxic to nontarget species. The success of spray- (Hoff et al. 1989, Mickle 1994), and a Bell 2o68 ing is driven by timing of the application and in Jetranger. Atomizers have included 11010 T-jets particular by the applicator, who ultimately is and wind-driven and high (14,000)-rpm electri- responsible for placing the pesticide into the tar- cally driven Micronair AU4OOO rotary atomiz- get area. Typically, aerial spraying for mosqui- ers. Simulants with physical properties similar toes incorporates an emission droplet size dis- to forestry insecticide formulations were used to tribution with a volume median diameter (D"o.r) produce representative emission droplet size dis- below 100 pm. Given the slow settling veloci- tributions. A computer-generated graphics pre- ties of these droplets, their initial motion is con- sentation of examples from both experiments trolled by the wingtip vortices of the spray air- can be obtained from the author. craft (Drummond 1987). Such small droplets The ARAL cloud mapper comprises a small have the disadvantage, however, that if they do Nd-Yag laser pulsing at 70 Hz and receiving op- not impact the target in a short time, they are tics to capture the returning signal. Under the highly susceptible to drift (Picot et al. 1986, control of a laptop computer, the laser beam is Crabbe and McCooeye 1989, Akesson et al. scanned through the spray cloud every 4 sec 1992, Crabbe et al. 1994). with a sweep taking slightly more than 2 sec. In order to diagnose the aerodynamics of the The time of flight of each laser pulse backscat- application technique, the Atmospheric Environ- tered from the droplets in the spray cloud gives ment Service (AES) of Environment Canada un- the range of the cloud from the ARAL, whereas dertook the construction of a lidar system (Hoff the intensity of the returning pulse is a measure et al. 1989, Mickle 1994) that was capable of of the droplet density within the reflecting vol- monitoring the spray drift cloud in real time. The ume of the cloud. Laser beam and receiving op- lidar system measures the intensity of the re- tics divergences permit volume sampling of I m3 turned laser pulse as a function of time (range) of the cloud at distances of I km from the thereby mapping the cloud density cross section ARAL system. The system is, therefore, capable as it moves away from the aircraft flight line. of clearly defining the spray cloud distribution From cross-sectional lidar profiles, it is possible within the wingtip vortices. to visualize the spray cloud application and, giv- Within the vortices (Fig. 1A), the cloud den- en the initial spray droplet size distribution and sity, as seen fTom a single laser pulse, was found a model for subsequent evaporation of the drop- to have 2 maxima of concentration, each asso- lets (Dumbauld et al. 1980, Picot et al. 1981, ciated with a center of the vortex pair. This dis- Mickle 1987, Teske et al. 1993), estimates of the tribution characterized the vast majority of the deposit and drift fractions can be calculated. operational atomizer configurations for ULV 372 373 JUNE1996 Syuposruu: Asnosot- Ct-ouo DvNeutcs 20 z E h15 t coREs tr i-"",:: oio J ul ts-5 J ul tr 0 1000 1100 {200 450 550 650 DISTANGEFROM ARAL (m) DISTANCEFROM ARAL (m) Fig. 1. Backscatter return from a single lidar pulse. A. TBM with 1O wind-driven AU4000 at lO,00O rpm. B. Bell 2O68 with 2 electrically driven AU4OOO at 14,000 rpm. spraying regardless of atomizer configuration or the differences between spraying in parallel and type. The example in Fig. 1A is for a TBM con- crosswinds. Selected cross sections of the spray figured with 5 AU40O0 atomizers mounted ap- cloud for parallel and crosswind cases are com- proximately I m below mid-chord of each wing. pared in Fig. 2 for various times after the aircraft The outermost atomizer was located at 567o of had passed a vertical plane swept by the laser the wing span. Despite the low mounting below beam. In both cases, the aircraft is flying away the wing, clearly the material has been moved from the observer (i.e., into the page) at a dis- spanwise towards the vortex core as the vortices tance of 1-2 km from the ARAL located on the were formed. No atomizers were mounted at the port side of the aircraft. Areas of equal droplet position of the vortex cores. The minimum be- density (concentration) are mapped with colors tween the peaks is associated with the lack of ranging from black (high concentration) to light atomizers below the center of the aircraft. It is gray (less than O.5Voof the high concentration). interesting that the shape of the droplet density A more detailed analysis of this case can be within the cloud is characteristic ofthe expected found in Mickle (1994). Figure 3 presents the deposit profile if an aircraft was flying close to time-integrated concentration (dosage) for these the ground. The only exception (Fig. 18) to this 2 spray cases at different heights and distances general shape was observed for a specialized relative to the flight line. Figure 4 presents the mounting of 2 AU40O0 atomizers near the cen- breathing height concentration of the spray with ter of a pallet slung approximately 3 m below a time, and Fig. 5 gives the accumulated deposit Bell 2068 Jetranger. In this case, the spray from from the loss of material in the surface layer. each atomizer formed an annulus around the Crosswind spray: In this example, a TBM vortex center at a distance characteristic of its with 5 Micronair AU4000 rotary atomizers lateral position from the aircraft center line. Al- (flow rate = 2 liters/min/head; D"o, : 4O-50 though material was found towards the center of pm) mounted under each wing was flown at a the vortex, clearly the contribution to spray height of 25 m above a clear-cut area. Winds at within the vortex core from atomizers at these aircraft height were light (1.8 m/sec) at 7O" to in-board locations is limited compared to the the flight line. Temperatures during this early more outboard locations as shown in the previ- morning stable spray run (0650 h AST) were ous example. near 9"C with relative humidity near 9O7o.With- in 9 sec after aircraft passage, the ULV spray CASE STUDIES had been swept into the wingtip vortices (Fig. Of the many flights that have been mapped, 2A) with the highest concentration (black) found the following 2 examples tend to characterize at the vortex centers. Within these 9 sec, the 374 JounNlr- or rtlg Avenlcat Mosquno CoNrnol AssocrerroN Vol. 12,No.2 TBM WITH AU4OOO TBM WITH 11010TJETS 50 z 100 o F E+o 9 SEC f, 980 3 SEC F z F30 F60 (,u z o 920 o40 o Irl U tv A =20 B F .! I 0 U 0 E z 50 o 100 F t g80 €oo 27 SEC F 27 SEC z E F30 o F60 -_-&- z I (920 (,o o40 q ITI =10 F =20 I U 0 c 0 50 100 €oo 69 SEC g80 ..1, 69 SEC F30 :s-'@'ttt F60 I __+-_ 'rS: I +1.;ls j o20 940 qMqF' 10 i{:,, ':,f' ul IIU - 20 0+ ,:{g:' 0 r050 1100 1150 1200 1250 {750 1800 1850 1900 1950 DISTANCEFROM LIDAR (m) DISTANCE FROM LIDAR (m) Fig. 2. Spray cloud concentration cross sections.A. Crosswind spray. B. Parallel wind spray. Contours representa 2-fold change in concentration. spray cloud had descended nearly lO m, which drift downwind, stretching longitudinally due to is characteristic of the vortex descent rate for the wind shear and with a vertical extent compara- TBM. Over the next 20-30 sec, the upwind vor- ble to the aircraft height. Concentrations within tex maintained its shape, carrying the spray from the cloud were typically less than lOVo of the the port wing as it impacted the ground approx- maximum concentration at 9 sec.
Details
-
File Typepdf
-
Upload Time-
-
Content LanguagesEnglish
-
Upload UserAnonymous/Not logged-in
-
File Pages8 Page
-
File Size-