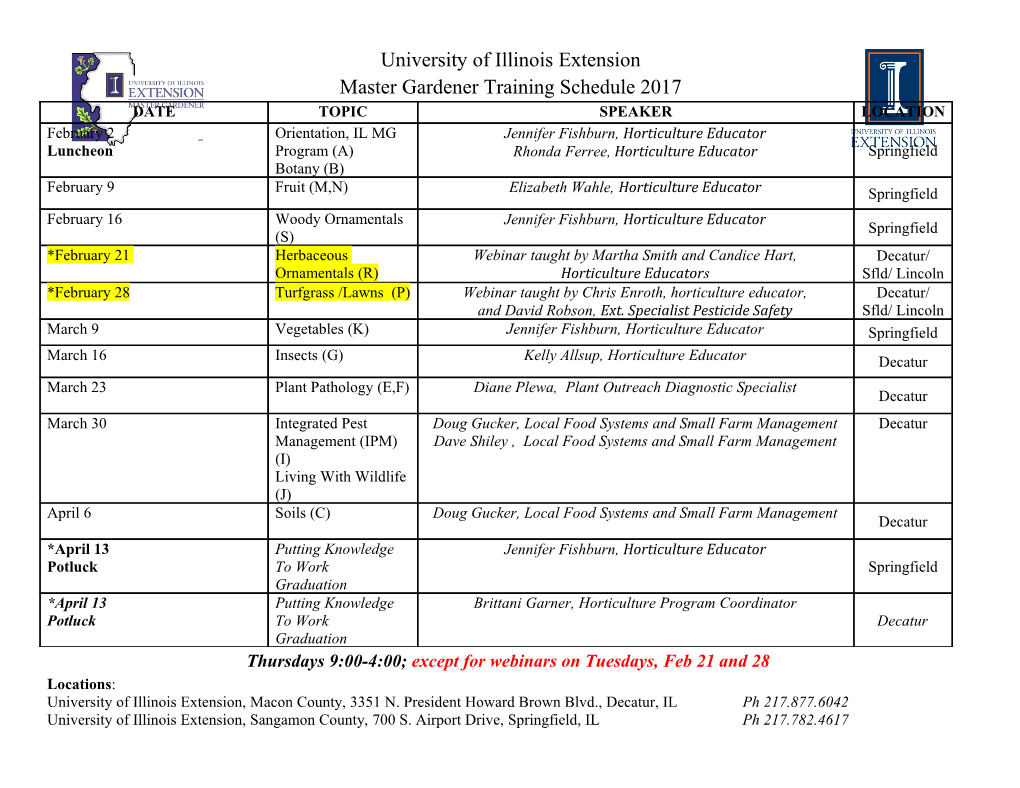
Alternative splicing in SCN1A: Biophysical consequences for NaV1.1 channels Emily Fletcher A thesis submitted to University College London for the degree of Doctor of Philosophy Department of Molecular Neuroscience Institute of Neurology Queen Square London WC1N 3BG 1 Declaration I, Emily Fletcher, confirm that the work presented in this thesis is my original research work. Where contributions of others are involved, this has been clearly indicated in the thesis. The copyright of this thesis rests with the author and no quotation from it or information derived from it may be published without the prior written consent of the author. 2 Acknowledgements I would like to thank my supervisor Stephanie Schorge for her continued support, patience and kindness towards me during my PhD and also to Dimitri Kullmann for the opportunity to work in his excellent lab. Most of all I‟d like to thank my family for their unconditional support and love. To my Dad for reading this gobbledygook through, to my Mum for her pep talks, to Ashleigh and my grandparents for making me laugh and to Claire especially, you‟re a fantastic big sister. Thank you. Lastly, I am truly grateful to have had opportunities for a good education. I am a very lucky person indeed. It's a new dawn It's a new day It's a new life For me And I'm feeling good Eunice Waymon, 1965 3 Abstract NaV1.1 is a voltage-gated sodium channel encoded by the gene SCN1A. Mutations in SCN1A cause dominantly inherited epilepsy syndromes in humans and NaV1.1 is an important target of several anti-epileptic drugs (AEDs). A common polymorphism in this gene has been shown to alter the expression of two splice variants of the channel, NaV1.1- 5N (containing exon 5N) and NaV1.1-5A (containing exon 5A). Although the splicing is highly conserved and the polymorphism that modifies it has been associated with altered AED dosage, the functional impact of the splicing on NaV1.1 is unknown. This project used whole cell voltage clamp of heterologously-expressed NaV1.1-5A and 5N to compare the intrinsic properties of the splice variants, their modulation by AEDs, their interaction with a published epilepsy mutation (R1648H), their modulation by G-proteins and how they responded to co-expression of sodium channel β subunits. The main finding was that, although when recorded at physiological temperatures the splice variants produced macroscopic currents that were similar for many parameters, they differed in the rate at which they recovered from inactivation, with NaV1.1-5N recovering more rapidly than NaV1.1-5A. This difference in recovery was conferred by a single amino acid substitution that is conserved in several sodium channels that are alternatively spliced at this site, and the difference was obscured in the presence of the common AED, phenytoin. Inclusion of the mutation R1648H also eradicated the difference by disproportionately slowing the recovery of NaV1.1-5N. Although several other subtle 4 differences were seen, no consistent differences were found in interactions between the splice variants and G-proteins or β subunits. By converging on a single parameter, recovery from inactivation, the data presented here suggest this parameter is modulated by splicing, and may play a role in development and treatment of seizures. 5 Table of Contents Chapter 1: Introduction .................................................................................................... 31 Overview of introduction ............................................................................................. 31 1.1.1 Original characterization and cloning of VGSCs ..........................................................33 1.1.2 NaV1.1 ...........................................................................................................................36 1.1.3 Structure of VGSC α subunits .......................................................................................37 1.1.4 Structural elements underlying channel gating .............................................................40 1.1.5 Structural elements underlying channel permeation .....................................................40 1.1.6 Inactivation of VGSCs ..................................................................................................41 1.1.7 The Persistent Sodium Current .....................................................................................42 1.1.8 Persistent sodium currents in disease ............................................................................45 1.1.9 A select overview of proteins that interact with VGSCs ..............................................46 1.1.10 Accessory β subunits ...................................................................................................48 1.1.11 β1 ................................................................................................................................50 1.1.12 β1B ..............................................................................................................................52 1.1.13 β2 ................................................................................................................................52 1.1.14 β3 ................................................................................................................................53 1.1.15 β4 ................................................................................................................................53 1.1.16 Proposed alternative functions of β subunits ..............................................................54 1.2.1 A selected overview of the historical and social impact of inherited epilepsies ...........56 1.2.2 Classification of seizures ..............................................................................................57 1.2.3 Epilepsy syndromes ......................................................................................................59 1.2.4 Diagnosis of epilepsy ....................................................................................................60 1.2.5 SCN1A and epilepsy .....................................................................................................63 1.2.6 GEFS+ is also caused by other ion channel related genes ............................................66 1.2.7 GEFS+ Type 1 SCN1B ..................................................................................................66 1.2.8 GEFS+ Type 3 GABRG2 ..............................................................................................67 1.2.9 GEFS+ Type 5 GABRD ................................................................................................70 1.2.10 GEFS+ Type 7 SCN9A ................................................................................................71 6 1.2.11 Unclassified GEFS+ genes..........................................................................................72 1.2.12 Genotype phenotype relationships for mutations in SCN1A .......................................74 1.2.13 Other genes providing phenocopies of SCN1A mutations ..........................................75 1.2.14 Mouse models of SMEI ..............................................................................................78 1.2.15 Mouse models of GEFS+ ............................................................................................81 1.2.16 The original NaV1.1-R1648H pedigree .......................................................................81 1.2.17 Functional consequences of R1648H in NaV1.1 channels expressed in heterologous cells ........................................................................................................................................83 1.2.18 The transgenic mouse carrying R1648H .....................................................................86 1.2.19 The R1648H knockin mouse .......................................................................................87 1.2.20 Merging conflicting data on functional effects of GEFS+ mutations with a mechanism of seizures ...........................................................................................................89 1.2.21 Interactions with other channels .................................................................................92 1.3.1 Overview of alternative splicing ...................................................................................96 1.3.2 Alternative splicing in SCN genes ..............................................................................101 1.3.3 Alternative splicing in VGSC genes during epilepsy..................................................104 1.3.4 Functional studies of SCNnA splice variants ..............................................................105 1.3.5 Biophysical consequences of disease causing mutations in SCNnA splice variants ..106 1.3.6 Alternative splicing in other VGSC regions ...............................................................107 1.3.7 Functional significance of VGSC splice variants .......................................................110 1.3.8 Alternative splicing GEFS+ syndromes ......................................................................110 1.3.9 AEDs and splicing ......................................................................................................111 1.3.10 Can alternative splicing affect AED efficacy? ..........................................................112 1.3.11 Mechanism of AED block on VGSCs ......................................................................116
Details
-
File Typepdf
-
Upload Time-
-
Content LanguagesEnglish
-
Upload UserAnonymous/Not logged-in
-
File Pages382 Page
-
File Size-