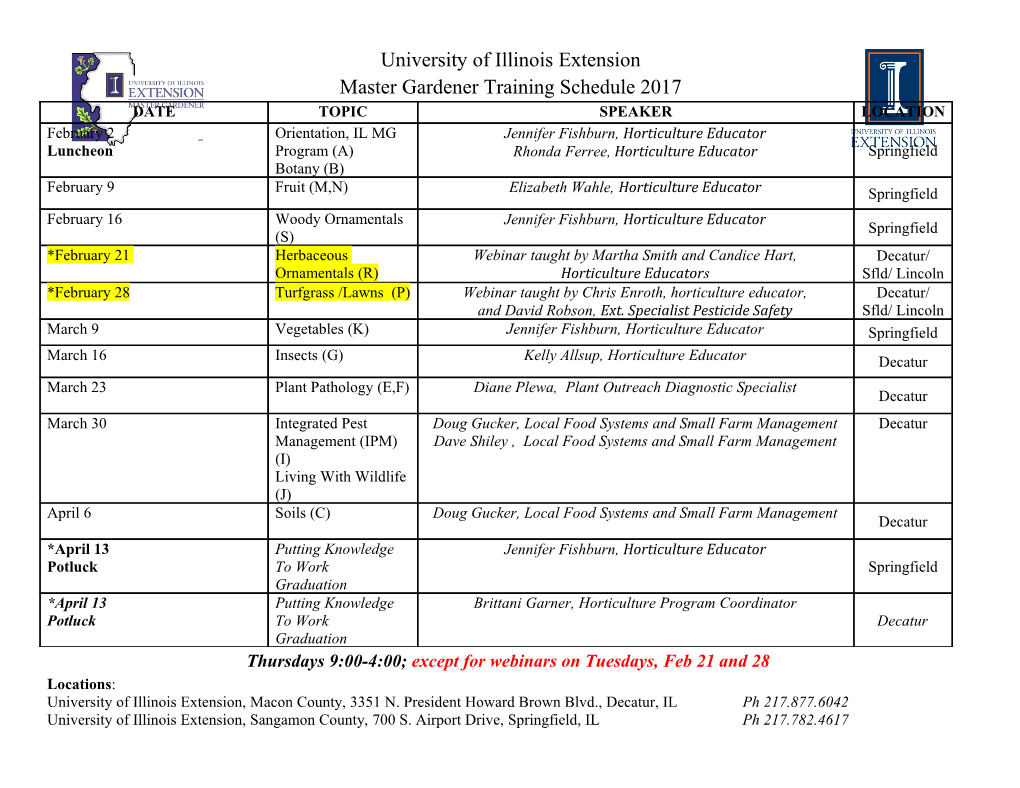
Femtosecond quantum control of molecular bond formation Patrick Nuernbergera,b,2, Daniel Wolperta, Horst Weissc, and Gustav Gerbera,1 aPhysikalisches Institut, Universität Würzburg, Am Hubland, 97074 Würzburg, Germany; bInstitut für Physikalische Chemie, Universität Würzburg, Am Hubland, 97074 Würzburg, Germany; and cPolymer Research Division, BASF SE, 67056 Ludwigshafen, Germany Edited* by Joshua Jortner, Tel Aviv University, Tel Aviv, Israel, and approved April 27, 2010 (received for review November 24, 2009) Ultrafast lasers are versatile tools used in many scientific areas, reaction pathways accessible by the short pulses and the corre- from welding to eye surgery. They are also used to coherently sponding nonequilibrium excitation of the substrate’s electronic manipulate light–matter interactions such as chemical reactions, system became apparent and revealed that femtosecond laser but so far control experiments have concentrated on cleavage or desorption is not only because of a phonon-assisted heating rearrangement of existing molecular bonds. Here we demonstrate effect. the synthesis of several molecular species starting from small reac- Besides desorption, nuclear wave-packet dynamics of adsorbed tant molecules in laser-induced catalytic surface reactions, and molecules could be manipulated (27, 28) by femtosecond lasers, even the increase of the relative reaction efficiency by feedback- and also a few chemical reactions could be induced. The most optimized laser pulses. We show that the control mechanism is prominent among them is the oxidation of carbon monoxide nontrivial and sensitive to the relative proportion of the reactants. in the presence of oxygen on various surfaces (29, 30). The The control experiments open up a pathway towards photocataly- pioneering work by Ertl and coworkers (30) beautifully revealed sis and are relevant for research in physics, chemistry, and biology the underlying reaction mechanism via hot substrate electrons, a where light-induced bond formation is important. mechanism that also accounts for the recombinative desorption of hydrogen under femtosecond laser irradiation, which clearly femtochemistry ∣ surface science differs from thermal excitation (31). However, only very few surface reactions could be observed or assisted by femtosecond ver since their invention, lasers were considered the ideal tool lasers, whereas complex catalytic reactions have been completely Efor microscopic control over chemical bonds, and several inaccessible up to now. seminal coherent control approaches have been developed We have chosen to explore the reaction of carbon monoxide (1–3). A very successful method to this task is femtosecond quan- and hydrogen, coadsorbed on a Pd(100) single crystal surface. tum control, where selectivity over photoinduced reactions is When adsorbed on Pd(100), CO does not decompose in the first achieved by exploiting the coherence properties and ultrashort layer and adsorbs bridge-bonded with the C atom binding to the time scales of femtosecond laser radiation (4–6). Combined with metal. If the molecular bond is broken [e.g., by electron bombard- learning algorithms processing experimental feedback to adap- ment (32)], the remaining C atoms can stay on the surface as tively find optimized pulses best suited for solving the control task bulk carbon, which lowers the adsorption energy for further (7), chemical reactions can even be controlled without a priori CO adsorption (33). H2 already adsorbs dissociatively at very knowledge about the reaction mechanisms. This scheme has been low coverages and subsequently penetrates into the bulk where successfully applied to dissociative reactions in the gas phase, it is dissolved (34). Because the maxima for thermal desorption first on organometallic compounds (8) and later on many other of hydrogen and CO are 360 (34) and 490 K (33), respectively, systems. The method is not limited to gas phase experiments, as experiments presented in this work have been performed at fluorescence optimizations of molecules in the liquid phase have 290 K, so that adsorbed species should stick well to the surface shown (9–12). Recently, also more complex control tasks have and their mobility be still quite high. Recently, coadsorption of been realized, like the energy flow in large biomolecules (13) or carbon monoxide and hydrogen on Pd(111) over a wide pressure the quantum yield in a photoisomerization reaction (14–16). Fem- range (35) revealed that high-pressure CO structures are identi- tosecond lasers have also been introduced to the field of photoas- cal to high-coverage structures in ultrahigh vacuum, but, despite sociation from atoms in cold traps, in both theory (17, 18) and first diverse gas amounts and compositions, no reaction product was experiments (19, 20). However, the selective laser manipulation of observed (35), presumably because of the high energy barriers. bond-forming reactions starting from small reactant molecules A femtosecond laser pulse can provide this energy, whereas spe- that may furthermore exhibit competing bond-forming reaction cially shaped pulses can steer the outcome into a desired reaction channels has not been shown yet. channel. In this contribution, we present the realization of femtosecond laser-assisted catalytic reactions of carbon monoxide and hydro- Experimental Setup gen or deuterium at a metal surface and further demonstrate that Our experiments are conducted with the setup displayed in Fig. 1. the relative reaction efficiency can be increased by the benefits of A femtosecond laser system delivers pulses that are phase- femtosecond laser pulses tailored especially for a desired reaction modulated in a LCD pulse shaper and then focused onto a metal outcome. These experiments represent a first step and a reaction path toward laser-induced catalysis of molecular systems. Author contributions: P.N., D.W., H.W., and G.G. designed research; P.N. and D.W. Femtosecond laser sources have been employed by laser performed research; P.N., D.W., H.W., and G.G. analyzed data; and P.N., D.W., H.W., scientists to explore processes on metal surfaces as soon as they and G.G. wrote the paper. were available. Other types of lasers have been used earlier for The authors declare no conflict of interest. this purpose, but starting from the first demonstration of intact *This Direct Submission article had a prearranged editor. desorption of NO molecules from a Pd(111) single crystal 1To whom correspondence should be addressed. E-mail: [email protected]. induced by femtosecond laser pulses (21), a complete field of 2Present address: Laboratoire d’Optique et Biosciences, Ecole Polytechnique, 91128 ultrafast laser spectroscopy on metal surfaces has emerged Palaiseau, France. among the diversity of surface chemistry techniques (22, 23). This article contains supporting information online at www.pnas.org/lookup/suppl/ Already in early time-resolved experiments (24–26) the unique doi:10.1073/pnas.0913607107/-/DCSupplemental. 10366–10370 ∣ PNAS ∣ June 8, 2010 ∣ vol. 107 ∣ no. 23 www.pnas.org/cgi/doi/10.1073/pnas.0913607107 Downloaded by guest on September 28, 2021 single mass spectra change, but also new ion signals arise with rising hydrogen concentration. New peaks at 13, 17, and 29 amu show up first, followed by peaks at 14, 15, 18, and 19 amu (Fig. 2, Green and Blue Lines). These peaks clearly indi- þ þ þ þ þ cate the formation of the ions CH ,CH2 ,CH3 ,OH ,H2O , þ þ H3O , and HCO . An analysis of the latter peak also indicates þ the formation of H2CO (Fig. S1). Varying the temperature of the single crystal from 190 to 390 K did not lead to any further ion peaks. No information can be derived whether methane ions (16 amu) are formed, because they would coincide with Oþ. The produced hydroxyl, water, and hydronium ion peaks emerge at the expense of the Oþ signal and are not because of residual water. By contrast, the production of methylidine, methylene, and methyl cations does not lead to a decrease of the Cþ signal, which actually grows with increasing H2 concentration. All ion Fig. 1. Experimental setup. The laser pulses are modulated in a LCD pulse shaper and then focused onto a Pd(100) single crystal surface located in signals fade away when the gas supply is stopped, with the hydro- the interaction region of a TOF-MS. The gases are dosed with mass flow con- gen peaks persisting longest. trollers and effusively stream via a nozzle and a skimmer onto the surface. The data are directly processed by a computer containing an evolutionary Single Parameter Variations. In order to further explore the algorithm. observed photochemical reactions, selected aspects of the macro- scopic experimental conditions have been changed. Hence, single crystal surface in a TOF-MS. Ions produced by the laser are additional information about the underlying mechanisms and detected in the TOF-MS, which is connected to a computer with a the role of the reactant molecules, the metal surface, and the learning algorithm. All presented experiments are performed at laser characteristics is obtained. room temperature and under high vacuum conditions. A more The assignment of the observed product ions is verified by detailed description is given in Materials and Methods. replacing H2 with D2 (Fig. 3). The ion peaks are separated by þ 2 amu, so that, e.g., the heavy water ion D2O appears at 20 amu. Results The relative efficiency of the bond-forming reactions is lower þ Experiments with Unshaped Pulses. When the laser beam does not compared to H2 as can, e.g., be seen by the weak D3O signal þ hit the surface, no ions are detected at all. As soon as H2 is and the absence of D2CO even for the strongest D2 excesses streamed onto the laser-irradiated surface, three huge peaks ap- employed (Fig. 3, Blue Line). This behavior can be interpreted þ þ þ pear in the ion spectrum, attributed to H ,H2 , and H3 (Fig. 2, as an indication for the reduced mobility of the heavier isotope Black Line).
Details
-
File Typepdf
-
Upload Time-
-
Content LanguagesEnglish
-
Upload UserAnonymous/Not logged-in
-
File Pages5 Page
-
File Size-