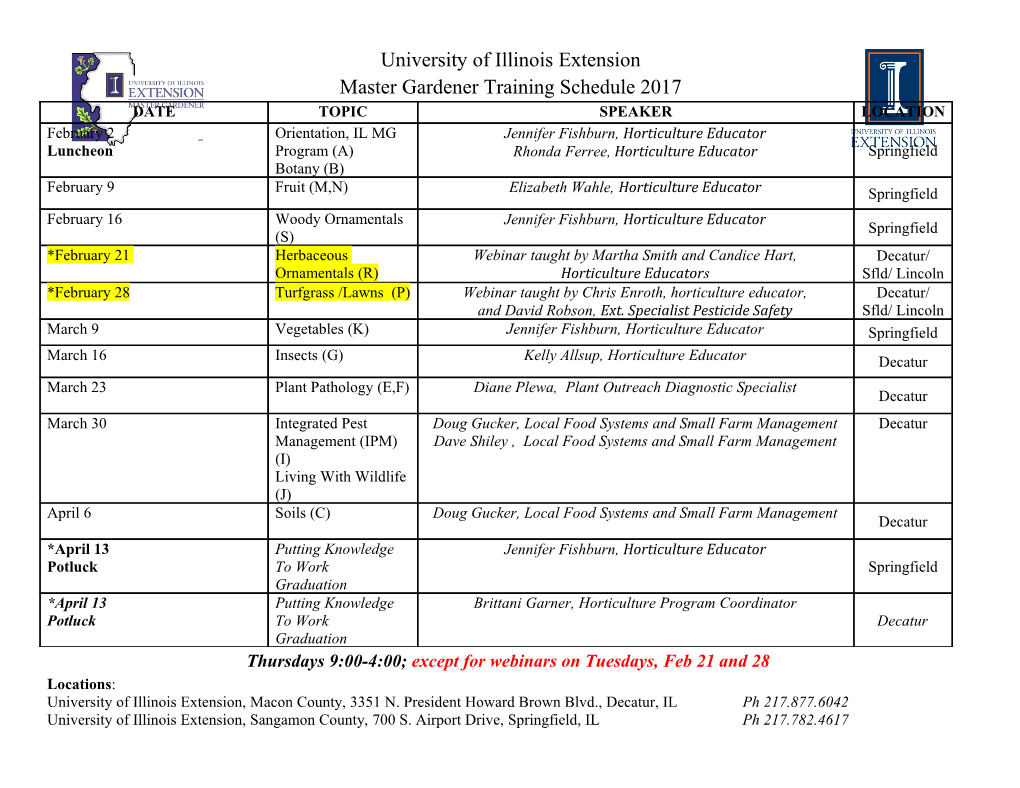
polymers Article Enhanced Electrorheological Response of Cellulose: A Double Effect of Modification by Urea-Terminated Silane Zhao Liu 1, Panpan Chen 1, Xiao Jin 1, Li-Min Wang 1, Ying Dan Liu 1,* and Hyoung Jin Choi 2,* ID 1 State Key Lab of Metastable Materials Science and Technology, College of Materials Science and Engineering, Yanshan University, Qinhuangdao 066004, Hebei, China; [email protected] (Z.L.); [email protected] (P.C.); [email protected] (X.J.); [email protected] (L.-M.W.) 2 Department of Polymer Science and Engineering, Inha University, Incheon 402751, Korea * Correspondence: [email protected] (Y.D.L.); [email protected] (H.J.C.) Received: 30 June 2018; Accepted: 2 August 2018; Published: 4 August 2018 Abstract: As a natural polymer with abundant sources, cellulose was one of the earliest applied electrorheological (ER) materials. However, cellulose-based ER materials have not attracted much attention because of their relatively low ER effect and sensitivity to water. In this study, cellulose rods were decorated with a urea-terminated silane, 1-(3-(trimethoxysilyl) propyl) urea, after being swelled in sodium hydroxide solution. The morphologies and structures of the cellulose particles were investigated using scanning electron microscopy, Fourier-transform infrared spectroscopy and X-ray diffraction, confirming the dramatic differences of the treated cellulose particles from the pristine cellulose. Rheological behaviors of the pristine and modified cellulose particles in silicone oil were observed using a rotational rheometer. It was found that the silane-modified cellulose showed higher ER effect and higher dielectric properties than the pristine cellulose particles, which was not only related to the grafted polar molecules but may also be associated with the porous morphologies of the treated cellulose particles. Keywords: electrorheological fluid; cellulose; swelling; silane; yield stress; dielectric spectra 1. Introduction Cellulose is the most abundant biopolymer, and comes from the cell wall of plants and bacteria, having an annual production of 1.5 × 1012 tons [1,2]. The current environmental and energy-related problems have been motivating the development of novel production approaches that are both sustainable and eco-friendly. As a renewable, biodegradable and inexpensive material, cellulose has attracted much attention since it was initially characterized in 1838 [3]. It is well known that cellulose is composed of three basic elements of C, H and O, being a typical carbohydrate. D-pyranose, which has three –OH groups as the repeating units linked by the glycosidic bond, forms the chain conformation with successive glucose rotated through an angle of 180◦ about the molecular axis and hydroxyl groups in an equatorial position [4–7]. The physical and chemical properties of cellulose are different from those of other polymers and are largely dependent on its supramolecular structure [8–11]. The supramolecular structure of cellulose is not simply based on the gathering of the six-membered rings held together by physical interaction [12]. Rather, the anhydroglucose units (AGUs) are linked by β-1,4-glycosidic linkages, a kind of covalent bond, considered to be the specific structure. The large cohesive energy in cellulose obviously reveals extensive hydrogen networks between intermolecular and intramolecular interactions formed by three –OH groups [13,14]. The ability of these hydroxyl groups to form hydrogen bonds plays a major role in the formation of fibrillar and semicrystalline packing, hence cellulose has at least five allomorphic forms [1,15–17]. Polymers 2018, 10, 867; doi:10.3390/polym10080867 www.mdpi.com/journal/polymers Polymers 2018, 10, 867 2 of 16 Both physical and chemical methods can be used to change the morphology and properties of cellulose. A high-pressure homogenization method [18,19] and ball-milling [20,21] are used to make the cellulose smaller and amorphous. Noncovalent modification [22], sulfonation [23], TEMPO-mediated oxidation [24], esterification [25–27], etherification [28] and silylation [29,30] are chemical approaches applied to change the properties of cellulose. These effective methods allow cellulose to have more extensive applications, such as in composite materials, optical films, pharmaceuticals and foodstuffs, as well as in the dispersed phase of electrorheological fluids [26,27,31]. Electrorheological (ER) fluids are a form of smart colloidal suspensions, whose rheological properties will change significantly upon the application of an electric field [32–34]. The ER fluids consist of dielectric or semiconducting particles as the dispersed phase and insulating liquids as the continuous phase. When an electrical field stimulus is applied, the dispersed particles are able to be polarized and form clusters, chains or column structures. This makes the ER fluids transform from a liquid-like state to a solid-like state possessing a typical yield stress. The rheological properties of an ER fluid, including flow curves of shear stress or shear viscosity and dynamic modulus, are mainly dependent on the field-induced structures formed by the dispersed particles. To date, a variety of inorganic and organic materials that have specific ER effects when dispersed in insulating liquids have been investigated. Some compounds are attracting attention, such as titanium oxide with different morphologies, and calcium and strontium titanates, because of their high dielectric polarizability. The yield stress of these kinds of inorganic-based ER fluids can be over 130 kPa or higher when modified by polar molecules [35–38]. The high yield stress is actually enough for applications, however, inorganics always result in serious abrasion to devices and have poor suspension stability. Polymer-based ER materials are attractive candidates as they can overcome some drawbacks of inorganics, but they have relatively lower ER effects. Chemical modification and composite methods have been applied to enhance the ER effects of polymer-based ER materials [39–42]. Cellulose particles, as well as other biopolymers such as starch and chitosan, have been applied as ER materials because of their electro-responsive polar groups [43–45]. However, the pristine forms of these biopolymers are very sensitive to water due to their hydroxyl groups, which reduces the thermal stability and long-term stability of the ER fluid. Therefore, anhydrous biopolymers were introduced via various chemical modification methods including phosphorate and carbamate esterification. Consequently, outstanding ER effects have not been observed from these cellulose esters and microcrystalline cellulose particles. In the current study, urea-terminated silane was applied to modify cellulose particles via a hydrolysis process [46], before which the cellulose particles were swelled in sodium hydroxide solution. It was found that the chemically treated cellulose particles were extremely different from pristine cellulose in both morphology and structure. Rheological analysis indicated that the modified cellulose showed higher ER effect, which was attributed to the porous morphologies and decorated urea groups caused by the chemical modification process. 2. Materials and Methods 2.1. Materials Cellulose powders (C6288) used in this study were purchased from Sigma Aldrich (Shanghai, China). 1-(3-(trimethoxysilyl) propyl) urea (T1915, TCI, Shanghai, China), silicone oil (viscosity: 50 cSt; density: 0.96 g/mL) (Beijing Hangping Guichuang Chemical Co., Ltd., Beijing, China), sodium hydroxide (NaOH, analytical purity) (Tianjin Kaitong Chemical Reagent Co., Ltd., Tianjin, China), and hydrochloric acid (HCl, analytical purity) (Tianjin Jindongtianzheng Chemical Reagent, Tianjin, China) were used without any further purification. 2.2. Preparation of Silane-Modified Cellulose (Cel-Si-Urea) 5.0 g of cellulose powders were firstly dispersed in an aqueous solution of NaOH (10 wt %) and the suspension was precooled to −13 ◦C in a refrigerator to make the cellulose particles swell in the alkali Polymers 2018, 10, 867 3 of 16 Polymers 2018, 10, x FOR PEER REVIEW 3 of 15 solution. Then, the suspension was stirred vigorously until the suspension became semitransparent. 0.1alkali mol/L solution. HCl solution Then, was the dropped suspension into thewas above stirred suspension vigorously until until the pHthe value suspension reached became 7.0. In this processsemitransparent. the gelatinous 0.1 mol/L cellulose HCl precipitated solution was and dropped the suspension into the above became suspension opaque white.until the Another pH value liquid reached 7.0. In this process the gelatinous cellulose precipitated and the suspension became opaque mixture was then prepared by adding 40 mL alcohol to 160 mL of deionized water, into which the white. Another liquid mixture was then prepared by adding 40 mL alcohol to 160 mL of deionized cellulose suspension was poured. 1.0 g of 1-(3-(trimethoxysilyl) propyl) urea, pre-hydrolyzed in a water, into which the cellulose suspension was poured. 1.0 g of 1-(3-(trimethoxysilyl) propyl) urea, small amount of water, was then dropped into the cellulose suspension. The suspension was stirred pre-hydrolyzed in a small amount of water, was then dropped into the cellulose suspension. The at room temperature for 4 h, then the precipitates were washed with deionized water several times suspension was stirred at room temperature for 4 h, then the precipitates were washed with anddeionized freeze-dried. water several A schematic times reactionand freeze
Details
-
File Typepdf
-
Upload Time-
-
Content LanguagesEnglish
-
Upload UserAnonymous/Not logged-in
-
File Pages16 Page
-
File Size-