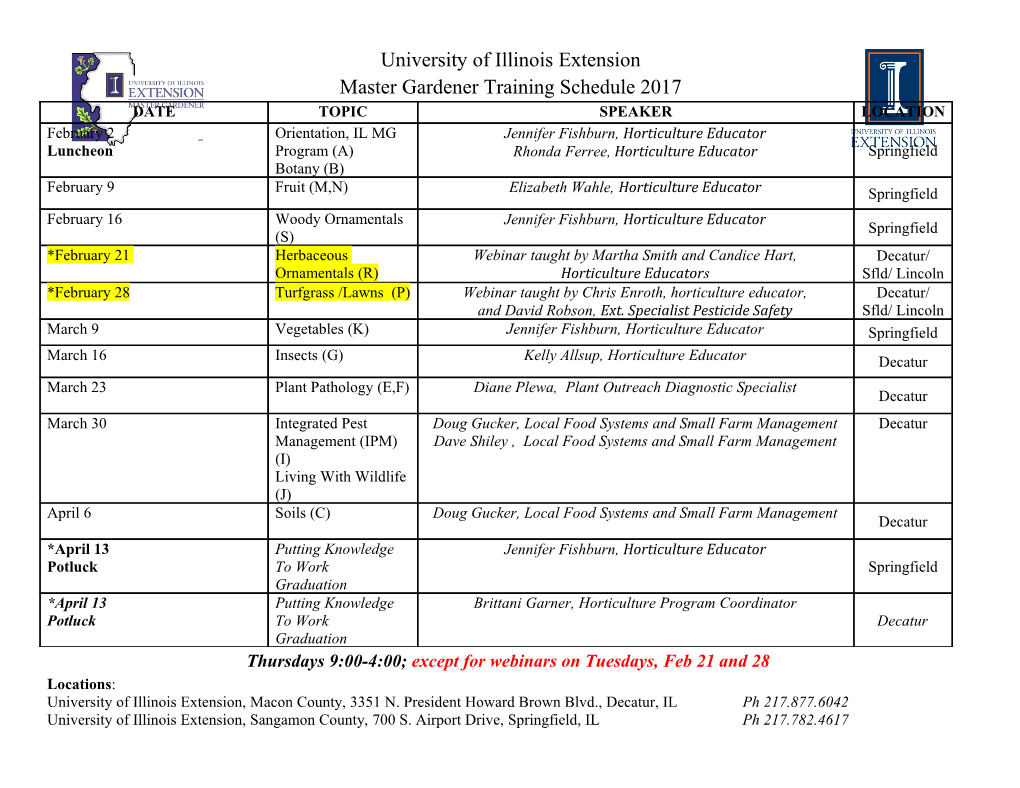
LINEAR MULTI-VARIABLE POLYLOGARITHMS Michał Zakrzewski Jan Kochanowski University, Kielce . [email protected] Będlewo, September 15th, 2015 Plan 1 Introduction Classical theory of polylogarithms and zeta-function Polylogarithm and Euler-Gauss hypergeometric function 2 Multi-variable polylogarithms Motivation. Shintani zeta-function and MZV Multiple zeta values General linear multi-variable polylogarithm 3 Multivariable hypergeometric functions and hypergeometric systems Multivariable hypergeometric functions GKZ systems Mordell-Tornheim GKZ-system Gen. D-K int. Bibliography More generally, the polylogarithmic series of order s is defined by X tn Li (t) := , |t| < 1. (2) s ns n>0 As re s > 1, function Lis is well defined on a closure D of the unit disc D and we have Lis (1) = ζ(s), where ζ is the famous Riemann zeta function. Classical polylogarithm The Dilogarithm function X tn Li (t) := , |t| < 1, (1) 2 n2 n>0 has been defined and extensively studied by Euler (mainly, but not only, in the article cited below). Euler, L., ”De summatione serierum in hac forma contentarum: a/1 + a2/4 + a3/9 + a4/16 + a5/25 + a6/36+ etc.” Memoires de l’academie des sciences de St.-Petersbourg 3, 1811, pp. 26 - 42. As re s > 1, function Lis is well defined on a closure D of the unit disc D and we have Lis (1) = ζ(s), where ζ is the famous Riemann zeta function. Classical polylogarithm The Dilogarithm function X tn Li (t) := , |t| < 1, (1) 2 n2 n>0 has been defined and extensively studied by Euler (mainly, but not only, in the article cited below). More generally, the polylogarithmic series of order s is defined by X tn Li (t) := , |t| < 1. (2) s ns n>0 Euler, L., ”De summatione serierum in hac forma contentarum: a/1 + a2/4 + a3/9 + a4/16 + a5/25 + a6/36+ etc.” Memoires de l’academie des sciences de St.-Petersbourg 3, 1811, pp. 26 - 42. Classical polylogarithm The Dilogarithm function X tn Li (t) := , |t| < 1, (1) 2 n2 n>0 has been defined and extensively studied by Euler (mainly, but not only, in the article cited below). More generally, the polylogarithmic series of order s is defined by X tn Li (t) := , |t| < 1. (2) s ns n>0 As re s > 1, function Lis is well defined on a closure D of the unit disc D and we have Lis (1) = ζ(s), where ζ is the famous Riemann zeta function. Euler, L., ”De summatione serierum in hac forma contentarum: a/1 + a2/4 + a3/9 + a4/16 + a5/25 + a6/36+ etc.” Memoires de l’academie des sciences de St.-Petersbourg 3, 1811, pp. 26 - 42. Operator θt λ satisfies the eigen-equation (θt − λ)t = 0. A power series and a Mellin transformation are spectral decompositions w. r. t. eigen-equation of θ. It follows, that the polylogarithmic function satisfies differential equation t θs Li (t) = , |t| < 1 (3) s 1 − t or, equivalently, s−1 (1 − t)∂t θt Lis (t) = 1, |t| < 1. (4) This differential equation, (3) or (4), can be engaged to analiticaly 1 continue Lis on C\{0, 1}, i.e. CP \{0, 1, ∞}. Classical polylogarithm. Differential equation Let ∂t := ∂/∂t and θ = θt = t∂t - the Euler operator. A power series and a Mellin transformation are spectral decompositions w. r. t. eigen-equation of θ. It follows, that the polylogarithmic function satisfies differential equation t θs Li (t) = , |t| < 1 (3) s 1 − t or, equivalently, s−1 (1 − t)∂t θt Lis (t) = 1, |t| < 1. (4) This differential equation, (3) or (4), can be engaged to analiticaly 1 continue Lis on C\{0, 1}, i.e. CP \{0, 1, ∞}. Classical polylogarithm. Differential equation Let ∂t := ∂/∂t and θ = θt = t∂t - the Euler operator. Operator θt λ satisfies the eigen-equation (θt − λ)t = 0. It follows, that the polylogarithmic function satisfies differential equation t θs Li (t) = , |t| < 1 (3) s 1 − t or, equivalently, s−1 (1 − t)∂t θt Lis (t) = 1, |t| < 1. (4) This differential equation, (3) or (4), can be engaged to analiticaly 1 continue Lis on C\{0, 1}, i.e. CP \{0, 1, ∞}. Classical polylogarithm. Differential equation Let ∂t := ∂/∂t and θ = θt = t∂t - the Euler operator. Operator θt λ satisfies the eigen-equation (θt − λ)t = 0. A power series and a Mellin transformation are spectral decompositions w. r. t. eigen-equation of θ. This differential equation, (3) or (4), can be engaged to analiticaly 1 continue Lis on C\{0, 1}, i.e. CP \{0, 1, ∞}. Classical polylogarithm. Differential equation Let ∂t := ∂/∂t and θ = θt = t∂t - the Euler operator. Operator θt λ satisfies the eigen-equation (θt − λ)t = 0. A power series and a Mellin transformation are spectral decompositions w. r. t. eigen-equation of θ. It follows, that the polylogarithmic function satisfies differential equation t θs Li (t) = , |t| < 1 (3) s 1 − t or, equivalently, s−1 (1 − t)∂t θt Lis (t) = 1, |t| < 1. (4) Classical polylogarithm. Differential equation Let ∂t := ∂/∂t and θ = θt = t∂t - the Euler operator. Operator θt λ satisfies the eigen-equation (θt − λ)t = 0. A power series and a Mellin transformation are spectral decompositions w. r. t. eigen-equation of θ. It follows, that the polylogarithmic function satisfies differential equation t θs Li (t) = , |t| < 1 (3) s 1 − t or, equivalently, s−1 (1 − t)∂t θt Lis (t) = 1, |t| < 1. (4) This differential equation, (3) or (4), can be engaged to analiticaly 1 continue Lis on C\{0, 1}, i.e. CP \{0, 1, ∞}. Sometimes it will be convenient to look at T in the context of formal series C[[tp]] in some power of the free variable. We have θTf = f , so we can regard T as a (right) inverse of θ. This leads to the integral representation t Li (t) = T s . (5) s 1 − t The domain of integration is the simplex in Rs . This is the simplest Drinfeld-Kontsevich integral. It will be introduced explicitly in the latter part of this talk. Classical polylogarithm. Integral R t Define the operator T as follows. Let Tf (t) := 0 f (τ)/τdτ, on tC[t]. In more general context, we understand T as a linear extension of the above oerator. We have θTf = f , so we can regard T as a (right) inverse of θ. This leads to the integral representation t Li (t) = T s . (5) s 1 − t The domain of integration is the simplex in Rs . This is the simplest Drinfeld-Kontsevich integral. It will be introduced explicitly in the latter part of this talk. Classical polylogarithm. Integral R t Define the operator T as follows. Let Tf (t) := 0 f (τ)/τdτ, on tC[t]. In more general context, we understand T as a linear extension of the above oerator. Sometimes it will be convenient to look at T in the context of formal series C[[tp]] in some power of the free variable. This leads to the integral representation t Li (t) = T s . (5) s 1 − t The domain of integration is the simplex in Rs . This is the simplest Drinfeld-Kontsevich integral. It will be introduced explicitly in the latter part of this talk. Classical polylogarithm. Integral R t Define the operator T as follows. Let Tf (t) := 0 f (τ)/τdτ, on tC[t]. In more general context, we understand T as a linear extension of the above oerator. Sometimes it will be convenient to look at T in the context of formal series C[[tp]] in some power of the free variable. We have θTf = f , so we can regard T as a (right) inverse of θ. The domain of integration is the simplex in Rs . This is the simplest Drinfeld-Kontsevich integral. It will be introduced explicitly in the latter part of this talk. Classical polylogarithm. Integral R t Define the operator T as follows. Let Tf (t) := 0 f (τ)/τdτ, on tC[t]. In more general context, we understand T as a linear extension of the above oerator. Sometimes it will be convenient to look at T in the context of formal series C[[tp]] in some power of the free variable. We have θTf = f , so we can regard T as a (right) inverse of θ. This leads to the integral representation t Li (t) = T s . (5) s 1 − t It will be introduced explicitly in the latter part of this talk. Classical polylogarithm. Integral R t Define the operator T as follows. Let Tf (t) := 0 f (τ)/τdτ, on tC[t]. In more general context, we understand T as a linear extension of the above oerator. Sometimes it will be convenient to look at T in the context of formal series C[[tp]] in some power of the free variable. We have θTf = f , so we can regard T as a (right) inverse of θ. This leads to the integral representation t Li (t) = T s . (5) s 1 − t The domain of integration is the simplex in Rs . This is the simplest Drinfeld-Kontsevich integral. Classical polylogarithm. Integral R t Define the operator T as follows. Let Tf (t) := 0 f (τ)/τdτ, on tC[t]. In more general context, we understand T as a linear extension of the above oerator. Sometimes it will be convenient to look at T in the context of formal series C[[tp]] in some power of the free variable. We have θTf = f , so we can regard T as a (right) inverse of θ.
Details
-
File Typepdf
-
Upload Time-
-
Content LanguagesEnglish
-
Upload UserAnonymous/Not logged-in
-
File Pages127 Page
-
File Size-