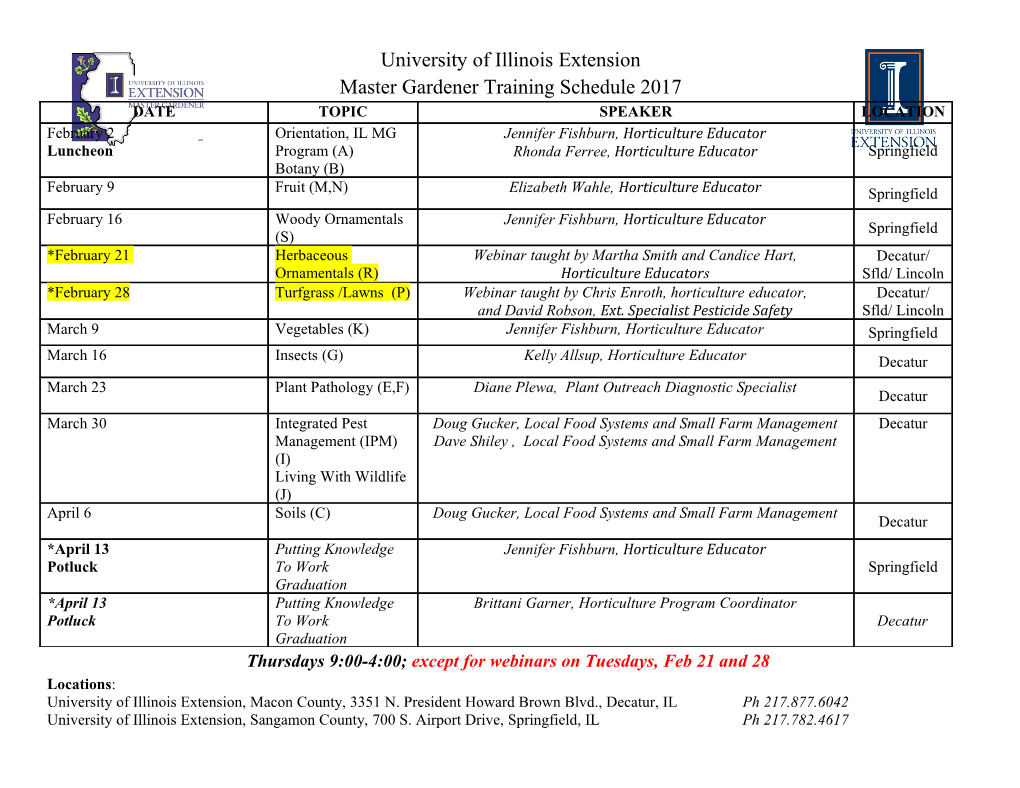
Electrosynthesis of Lithium Borohydride from Trimethyl Borate and Hydrogen Gas By James Mokaya Omweri Submitted in Partial Fulfillment of the Requirements For the Degree of Master of Science In the Chemistry Program YOUNGSTOWN STATE UNIVERSITY December, 2019 Electrosynthesis of Lithium Borohydride from Trimethyl Borate and Hydrogen Gas James Mokaya Omweri I hereby release this thesis to the public. I understand that this thesis will be made available from the OhioLINK ETD Center and the Maag Library Circulation Desk for public access. I also authorize the University or other individuals to make copies of this thesis as needed for scholarly research. Signature: James Mokaya Omweri, Student Date Approvals: Dr. Clovis A. Linkous, Thesis Advisor Date Date Dr. Sherri Lovelace-Cameron, Committee Member Date Dr. Timothy Wagner, Committee Member Date Dr. Salvatore A. Sanders, Dean of Graduate Studies Date ABSTRACT Solid state hydrogen storage is seen as the ultimate answer in realizing the hydrogen economy and minimizing our overdependence on nonrenewable fossil fuels. The use of fossil fuels has contributed negatively towards climate change, leading to a lot of future uncertainties. Alkali metal borohydrides, especially lithium borohydride, with desirable H2 storage properties such dry air stability and high gravimetric and volumetric storage densities of 18.5 wt% and 121 kg/m3, respectively, are proving to be some of the most important solid state hydrogen storage materials. However, their current cost of production is very high considering that most borohydrides are synthesized from sodium borohydride, which in turn is made from sodium hydride, NaH, and trimethyl borate, B(OCH3)3. NaH, which is a product of an energetic process, namely hydrogenation following electrodeposition of the metal from a molten salt, acts as the source of H- required for the formation of the borohydride ion. In this work, electrochemical transfer of H- from a Pd foil, which is considered less energetic than NaH, was investigated. Hydrogen gas at a pressure of about 1 atm was passed through the Pd foil, which was used as the working electrode in an electrochemical cell containing 0.1 M LiClO4 and 4.4 M B(OCH3)3 in CH3CN as the electrolyte. Using a potentiostat, a voltammetric experiment with 3 cycles at 50 mV/s was performed with and without hydrogen application. A potentiostatic experiment was conducted by holding the Pd foil at -2.75 V vs Ag/AgClO4 and run for 10.6 hours. Analysis of a portion of the electrolyte using IR and NMR spectrometry showed the presence of borohydride. A two compartment cell with Nafion as the separator of the electrode reagents was used to increase the yield. iii ACKNOWLEDGEMENT In a very special way, I would like to acknowledge my supervisor, Dr. Clovis A. Linkous for: accepting me to work and deliver this thesis in his research lab, supervising my work, his availability, patience, motivation, experience and immense knowledge on this area and all the thesis writing skills that he imparted on me. Dr. Linkous’s chronological order of knowledge presentation from simple to complex was very educative, thank you. Many thanks to Dr. Lovelace Cameron for her time, guidance and every time assurance that all will be well. Thank you for taking your time reading through the draft document and proposing the right corrections. Special thanks to Dr. Timothy Wagner for having time to go through my thesis draft and all the positive criticism on this research. I also thank Mr. Ray for helping in instrumentation. I would like to expand my sincere thanks to my lab colleagues: Jebet, Patrick, Milica, Kim, Linda and Solita for all the meaningful discussions. Last but not least, I would like to thank and appreciate my parents, family and close friends for their encouragement. Thank you all. iv Table of Contents ABSTRACT……………………………………………………………………………iii ACKNOWLEDGEMENT………………………………………………………………iv LIST OF FIGURES……………………………………………………………………..xi LIST OF TABLES………………………………………………………………….......xi 1.1 Introduction……………………………………………………………………….....1 1.2 Hydrogen gas as a green energy carrier……………………………………………..4 1.3 Hydrogen storage technologies………………………………………………………9 1.4 Hydrogen storage methods…………………………………………………………12 High pressure gas cylinders………………………………………………………….12 Liquid state hydrogen storage………………………………………………………..13 Solid state hydrogen storage methods……………………………………………….14 Carbon-based materials through physisorption………………………………………16 Hydrides……………………………………………………………………………...18 Metal hydrides……………………………………………………………………….18 Complex hydrides……………………………………………………………………21 Alanates……………………………………………………………………………23 Imides and amides…………………………………………………………………23 1.5 Borohydrides……………………………………………………………………….24 Sodium borohydride, NaBH4……………………………………………………...25 Lithium Borohydride, LiBH4……………………………………………………...27 1.6 Objective……………………………………………………………………………31 1.7 Hypothesis………………………………………………………………………….31 1.8 Significance of the study…………………………………………………………...32 Chapter 2: Instrumentation, materials and experimental methods……………………..33 v 2.1 Electrochemical Methods…………………………………………………………..33 Potentiostat…………………………………………………………………………...33 Electrochemistry……………………………………………………………………..34 Cyclic voltammetry…………………………………………………………………..36 Potentiostat experiments……………………………………………………………..41 The two-compartment cell…………………………………………………………...45 The Nafion membrane……………………………………………………………….45 2.2 Infrared spectroscopy………………………………………………………………48 2.3 Proton NMR………………………………………………………………………..51 Chapter 3: Results and discussion……………………………………………………..52 Chapter 4: Conclusion and future work……………………………………………….80 References……………………………………………………………………………...82 vi List of Figures Figure 1: World primary energy consumption……………………………………………2 Figure 2: Comparison of gravimetric and volumetric energy densities of selected fuels ..5 Figure 3: Proton Exchange Membrane fuel cell…………………………………………..6 Figure 4: Setup for a fuel cell vehicle……………………………………………………..7 Figure 5: The hydrogen fuel cycle ………………………………………………………..8 Figure 6: Hydrogen storage methods ……………………………………………………10 Figure 7: Hydrogen phase diagram ……………………………………………………...11 Figure 8: Volumetric representation of equal masses of hydrogen gas stored via different methods ………………………………………………………………………………….14 Figure 9: Stored hydrogen gas in different materials ……………………………………15 Figure 10: Schematic diagrams for chemisorption and physisorption …………………..17 Figure 11: Energies involved in chemisorption and physisorption of hydrogen gas onto metal surfaces…………………………………………………………………………….19 Figure 12: Van’t Hoff plots of some selected hydrides …………………………………21 Figure 13: Maximum deliverable hydrogen from hydrolysis of ionic hydrides ………...22 Figure 14: Brown and Schlesinger process for synthesis of NaBH4 …………………...26 Figure 15: Schematic diagram of the proposed study……………………………………31 Figure 16: A basic potentiostat…………………………………………………………..33 Figure 17: Cyclic voltammogram showing oxidation and reduction processes after scan ……………………………………………………………………………………………37 Figure 18: An electrochemical cell for cyclic voltammetry …………………………….37 vii Figure 19: Effect on potential window for different solvents, electrodes and supporting electrolytes ………………………………………………………………………………38 Figure 20: Electrochemical cell components…………………………………………….42 Figure 21: Experimental set-up for cyclic voltammetry…………………………………44 Figure 22: Two compartment cell assembly……………………………………………..45 Figure 23: Nafion membrane…………………………………………………………….45 Figure 24: CV experimental set-up for ferrocene,……………………………………… 47 Figure 25: IR absorption bands ………………………………………………………….49 Figure 26: FT-IR spectrophotometer ……………………………………………………50 Figure 27: IR spectra of reaction product sodium borohydride (A) and that of pure sodium borohydride, (B) ………………………………………………………………...50 Figure 28: Cyclic voltammogram for 1 M H2SO4 using palladium foil as the working electrode………………………………………………………………………………….52 Figure 29: Cyclic voltammogram for 0.1 M LiClO4 + CH3CN………………………….53 Figure 30: Reference electrode potential of ferrocene/silver wire……………………….54 Figure 31: Cyclic voltammogram for 0.05 M LiClO4 + CH3CN + 4.4 M TMB………...55 Figure 32: Cyclic voltammogram for 0.1 M LiClO4 + CH3CN + 4.4 M TMB + H2 …...56 Figure 33: An overlay of voltammograms showing the effect of H2 and TMB…………56 Figure 34: Potentiostatic experiment, WE electrode held at -2.75 V, + H2 at a pressure for 2 hours………………………………………………………………………………..57 Figure 35: IR spectra of ammonium hydroxide solution………………………………...58 Figure 36: IR spectra of 0.5 M lithium borohydride in ammonium hydroxide………...59 viii Figure 37: IR spectra of 0.5 M sodium trimethoxy borohydride in ammonium hydroxide………………………………………………………………………………...59 Figure 38: IR spectrum of the electrolyte (0.05 M LiClO4 + CH3CN + 4.4 M TMB) before electrolysis………………………………………………………………………..60 Figure 39: Expanded IR spectrum of the electrolyte (0.05 M LiClO4 + CH3CN + 4.4 M TMB) before electrolysis………………………………………………………………...61 Figure 40: IR spectrum of 0.05 M LiClO4, 4.4 M TMB in CH3CN after 2- hour electrolysis at -2.75 V vs Ag/AgClO4 under H2 pressure……………………………….62 Figure 41: Proton nmr of 0.1 M LiClO4 + CH3CN + 4.4 M TMB………………………63 Figure 42: Proton nmr spectrum of the 2 h. electrolysis product, including H2, TMB, LiClO4 and CH3CN………………………………………………………………………63 Figure 43: Cyclic voltammogram for 40 mM methanol on Pd foil in LiClO4/CH3CN….64 Figure 44: IR spectrum of 0.1M NaBH (OCH3)3 in ammonium hydroxide…………….66 Figure 45: IR spectrum of 0.5 M NaBH(OCH3)3 in ammonium hydroxide……………..66 Figure 46: Cyclic voltammogram
Details
-
File Typepdf
-
Upload Time-
-
Content LanguagesEnglish
-
Upload UserAnonymous/Not logged-in
-
File Pages98 Page
-
File Size-