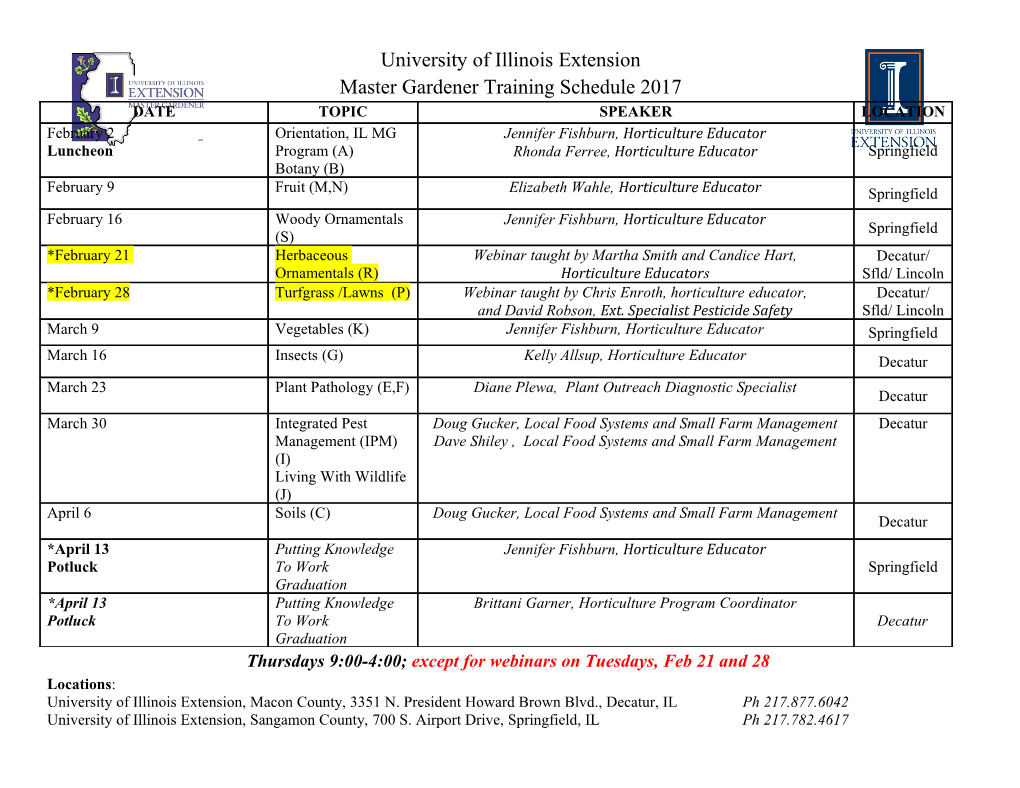
Digital Comprehensive Summaries of Uppsala Dissertations from the Faculty of Science and Technology 1947 Bacterial DNA repair and molecular search ARVID H. GYNNÅ ACTA UNIVERSITATIS UPSALIENSIS ISSN 1651-6214 ISBN 978-91-513-0966-8 UPPSALA urn:nbn:se:uu:diva-410039 2020 Dissertation presented at Uppsala University to be publicly examined in A1:111a, BMC, Husargatan 3, Uppsala, Friday, 28 August 2020 at 09:00 for the degree of Doctor of Philosophy. The examination will be conducted in English. Faculty examiner: Professor Stephen Kowalczykowski (University of California, Davis). Abstract Gynnå, A. H. 2020. Bacterial DNA repair and molecular search. Digital Comprehensive Summaries of Uppsala Dissertations from the Faculty of Science and Technology 1947. 77 pp. Uppsala: Acta Universitatis Upsaliensis. ISBN 978-91-513-0966-8. Surveillance and repair of DNA damage is necessary in all kinds of life. Different types of DNA damage require different repair mechanisms, but these mechanisms are often similar in all domains of life. The most serious type of damage, double stranded DNA breaks, are for example repaired in conceptually similar ways in both bacteria and eukaryotes. When this kind of breaks are repaired by homologous recombination, a homology to the site of the break must be found. Sometimes, this homology can be located far away from the break necessitating a search. Considering the large amount of heterologous DNA present, the complexity of this search is enormous. If and how this search can proceed has been unclear even in simple and well characterized organisms as E. coli. In this thesis, microscopy together with microfluidics are used to show that DNA repair by homologous recombination occurs even between homologies separated by several micrometers. We also see that it finishes well within the time of a cell generation, with the enigmatic search phase being as quick as eight or possibly even three minutes. Since this time is much faster than expected, we present a physical model demonstrating how homology search on this time scale is indeed plausible. Based on these results, we conclude that homologous repair using distantly located templates is likely to be a physiologically relevant mechanism of DNA repair. Microscopy together with image analysis by deep learning also provides a new method of detecting DNA damage in real time. Combined with tracking of cell lineages, it reveals that DNA damage in E. coli is repaired efficiently enough that the resulting fitness cost is close to none. With the same methods we also study the effect of deletions of several DNA repair enzymes, and largely confirms their previous characterizations. Among these, we confirm that the intriguing RecN protein is important but not absolutely necessary in DSB repair, that it acts early, and possibly aids in physically shaping the structure mediating the search. In addition to this, it is shown how DNA transcription and translation modulates the shape of the E. coli nucleoid. We observe how strong a transcription of a gene within a few minutes moves the gene towards the periphery of the cell where the concentration of ribosomes is higher, a movement possibly also aided by protein translation. We also present MINFLUX, a microscope for both nanometer scale localization of single fluorophores as well as in vivo single particle tracking with unprecedented trace length and resolution. Using this, the E. coli small ribosomal subunit could be observed to quickly shift between fast and slow diffusion states which might represent probing and discarding of RNAs suitable for translation. Keywords: DNA repair, DNA damage, homologous recombination, homologous repair, recombination, RecA, I-SceI Arvid H. Gynnå, Department of Cell and Molecular Biology, Molecular Systems Biology, Box 596, Uppsala University, SE-751 24 Uppsala, Sweden. © Arvid H. Gynnå 2020 ISSN 1651-6214 ISBN 978-91-513-0966-8 urn:nbn:se:uu:diva-410039 (http://urn.kb.se/resolve?urn=urn:nbn:se:uu:diva-410039) Ett hisnande faktum är att det till stora delar är samma DNA-molekyl som ger upphov till olika organismer, vare sig de är bakterier, växter, djur eller människor. Nationalencyklopedin, 1991 List of Papers This thesis is based on the following papers, which are referred to in the text by their Roman numerals. I Arvid H. Gynnå*, Jakub Wiktor*, Prune Leroy, Johan Elf. (2020) RecA mediated homology search finds segregated sister locus in minutes after a double stranded break. bioRxiv 2020.02.13.946996; Manuscript. II Sora Yang*, Seunghyeon Kim*, Dong-Kyun Kim, Hyeong Jeon An, Jung Bae Son, Arvid H. Gynnå, and Nam Ki Lee. (2019) Transcription and Translation Contribute to Gene Locus Reloca- tion to the Nucleoid Periphery in E. Coli. Nature Communica- tions 10 (1): 1–12. III Francisco Balzarotti*, Yvan Eilers*, Klaus C. Gwosch*, Arvid H. Gynnå, Volker Westphal, Fernando D. Stefani, Johan Elf, and Stefan W. Hell. (2017). Nanometer Resolution Imaging and Tracking of Fluorescent Molecules with Minimal Photon Fluxes. Science 355 (6325): 606–12. Reprints were made with permission from the respective publishers. Other works not included in the thesis: iv A. Sanamrad, F. Persson, E. G. Lundius, D. Fange, A. H. Gynnå and J. Elf. (2014). Single-Particle Tracking Reveals That Free Ribosomal Subunits Are Not Excluded from the Escherichia Coli Nucleoid. PNAS 111 (31): 11413–18. v J. Liljeruhm, ..., A. C. Forster. (2018) Engineering a Palette of Eukaryotic Chromoproteins for Bacterial Synthetic Biology. Journal of Biological Engineering 12 (May): 8. vi C. Vogel, A. H. Gynnå, J. Yuan, L. Bao, J. Liljeruhm, A. C. Forster. (2020) Rationally designed Spot 42 RNAs with an inhi- bition/toxicity profile advantageous for engineering E. coli. En- gineering Reports. 2020;e12126. vii M. Stårsta, D. L. Hammarlöf, M. Wäneskog, S. Schlegel, F. Xu, A. H. Gynnå, M. Borg, S. Herschend, and S. Koskiniemi. (2020). RHS-Elements Function as Type II Toxin-Antitoxin Modules That Regulate Intra-Macrophage Replication of Salmo- nella Typhimurium. PLoS Genetics 16 (2): e1008607. Contents Introduction ................................................................................................... 11 Types of DNA damage and repair ................................................................ 13 Causes and types of double stranded breaks ............................................ 14 Repair of double stranded breaks ............................................................. 16 Homologous recombination repair ........................................................... 17 The actors of homologous recombination repair ...................................... 19 Endonuclease RecBCD ........................................................................ 19 Recombinase RecA .............................................................................. 23 Double stranded breaks and molecular search ............................................. 28 Creating double stranded breaks .............................................................. 28 Detection of DSBs .................................................................................... 29 Inducing double stranded breaks in microfluidics.................................... 31 RecA activity after a double stranded break............................................. 35 Superresolution imaging ...................................................................... 37 Double stranded breaks and the cell cycle .................................................... 40 Detecting DNA damage by a neural net classifier ................................... 41 Effects of spontaneous DNA damage ...................................................... 42 Early and late participants in homologous repair .......................................... 44 Dynamics of chromosomal loci .................................................................... 52 Active single particle tracking ...................................................................... 54 Tracking results ........................................................................................ 55 Discussion ..................................................................................................... 57 Search time of homologous recombination .............................................. 59 How long is the search time actually? ...................................................... 60 After homology search ............................................................................. 61 The penalty of DNA damage.................................................................... 62 Conclusion and outlook ............................................................................ 63 Sammanfattning på svenska .......................................................................... 65 Acknowledgements ....................................................................................... 67 References ..................................................................................................... 69 Abbreviations ATC Anhydrotetracycline, a tetracycline analogue BER Base excision repair DNA Deoxyribonucleic acid DSB Double stranded break DSE Double stranded end dsDNA Double stranded DNA FWHM Full width at half maximum HR Homologous recombination HRR Homologous recombination repair HJ Holliday junction IPTG Isopropyl β-d-1-thiogalactopyranoside, a lactose analogue NER Nucleotide excision repair NHEJ Non-homologous end joining PSF Point spread function RESOLFT Reversible saturable optical fluorescence transitions RNA Ribonucleic
Details
-
File Typepdf
-
Upload Time-
-
Content LanguagesEnglish
-
Upload UserAnonymous/Not logged-in
-
File Pages78 Page
-
File Size-