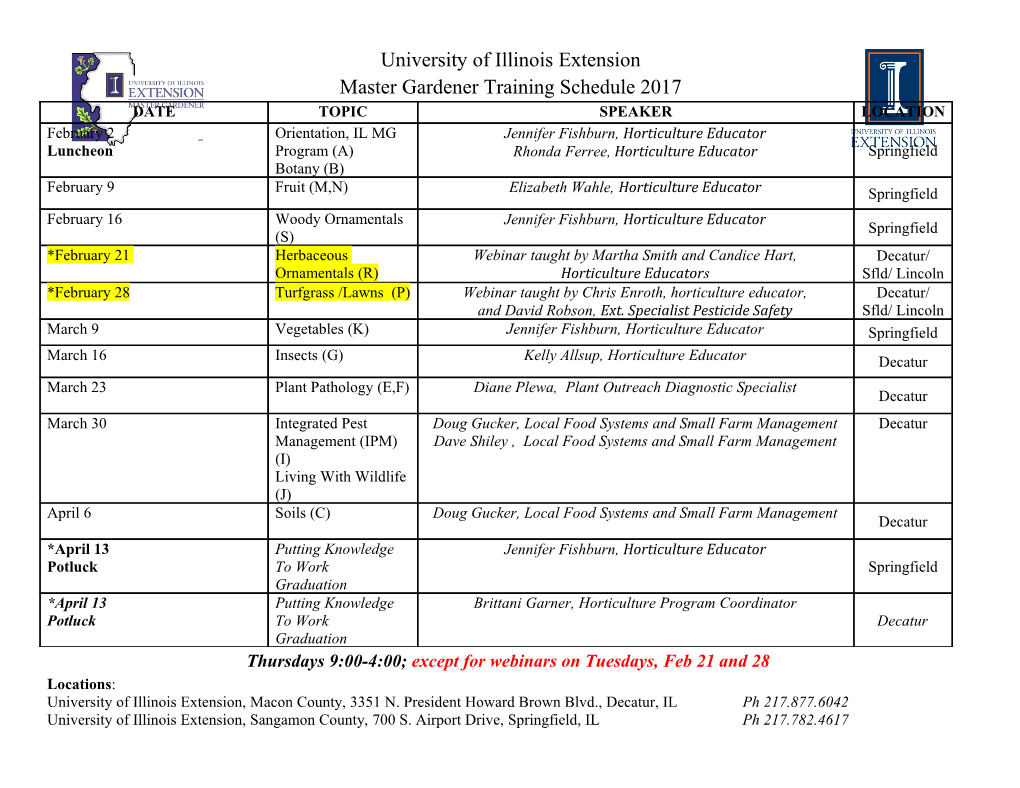
A review of future experiments Albrecht Karle University of Wisconsin-Madison Neutrino 2012 Kyoto Outline: •Neutrinos and Cosmic rays •Energy scales of neutrino telescopes, next challenges •1 to 100 GeV: Low energy extensions: PINGU,... •0.1 to 10000 TeV: Neutrino telescopes for neutrino astronomy •10^16 to 10^20eV: Strategies for cosmogenic neutrino flux discovery Albrecht Karle, UW-Madison Cosmic Rays and Neutrino Sources : neutrinos from accelerators Can neutrinos reveal origins Cosmic rays of cosmic rays? T. Gaisser 2005 pγ → pπ 0 ,nπ + + + π → µ + νµ + + µ → e + νe + νµ Cosmic ray interaction in accelerator region knee Prime Candidates 1 part m-2 yr-1 – SN remnants – Active Galactic Nuclei Ankle – Gamma Ray Bursts 1 part km-2 yr-1 2 Neutrino producUon from cosmic rays on known targets. T. GaisserT. Gaisser 2005 2005 pp → NN + pions; pγ → pπ 0 ,nπ + + + π → µ + νµ + + µ → e + νe + νµ Known targets: • Earth’s atmosphere: Atmospheric neutrinos (from π and K decay) • Interstellar maer in GalacUc plane: Cosmic rays interacUng with Interstellar maer, concentrated in the disk • Cosmic Microwave background: UHE cosmic rays interact with photons in intergalacUc photon fields. 3 Neutrino producUon from cosmic rays on known targets. T. GaisserT. Gaisser 2005 2005 pp → NN + pions; pγ → pπ 0 ,nπ + + + π → µ + νµ + + µ → e + νe + νµ Known targets: • Earth’s atmosphere: Atmospheric neutrinos (from π and K decay) Atmospheric neutrinos: • Interstellar maer in GalacUc AMANDA, IceCube plane: Cosmic rays interacUng with Interstellar maer, concentrated in the Waxman –bahcall upper bound disk IceCube E^-2 upper limit • Cosmic Microwave background: Cosmogenic UHE cosmic rays interact with GalacUc neutrino neutrino flux, flux model: eg ESS (blue line) photons in intergalacUc photon eg. Ingelman & Thunman fields. 4 How to detect UHE high energy neutrinos? The challenge: • Fluxes are small • The cross secUon is small Need to instrument/view very large target mass • backgrounds from cosmic rays, cosmic ray muons are high Need some overburden (or other good discriminaon) • Need to use natural targets, which are free, but – need to deal with environmental challenges – no control of the medium – lack of infrastructure (access, power, communicaons) – possibly unstable backgrounds Challenges for Calibraon full understanding of the medium, noise backgrounds, sensiUvity of sensor in situ (absolute sensiUvity, angular response) 5 Water/ice Cherenkov detectors: IceCube IceCube • Total of 86 strings and 162 IceTop tanks; • Compleon with 86 strings: December 2010 • Full operaon with all strings since May 2011. For results: see talks by G. Sullivan and A. Ishihara and numerous posters at this conference 6 A. Karle, UW-Madison 6 Water/ice Cherenkov detectors: Neutrino effecUve areas Wide energy range due to increase in effec1ve area! Area at 100 TeV (1TeV) 5 2 2 ) 10 IceCube 86: 40m (0.3m ) 2 10 4 Deep Core lowers threshold 3 10 from 100 GeV to 10 GeV. 10 2 10 IC-80 up TL EffecUve area for 1 νµ -1 Strong rise with energy: 10 – (up to 100TeV) -2 Antares 2007-8 10 – Increase of muon range Effective Neutrino Area (m -3 with energy up to PeV 10 – Flaening above PeV -4 energies. 10 IC-79 all sky PS -5 10 -6 10 1 2 3 4 5 6 7 8 9 10 11 log10(Primary Neutrino Energy - GeV) 7 Water/ice Cherenkov detectors: Neutrino effecUve areas Energy scales and future detectors - from low to high energy. PINGU: lower threshold 5 ) 10 from ~10 to few GeV 2 10 4 10 3 10 2 10 IC-80 up TL 1 -1 10 -2 Antares 2007-8 10 Effective Neutrino Area (m -3 10 -4 10 IC-79 all sky PS -5 10 -6 10 1 2 3 4 5 6 7 8 9 10 11 log (Primary Neutrino Energy - GeV) Low energy extensions 10 to IceCube’s DeepCore: PINGU, MICA 8 Water/ice Cherenkov detectors: Neutrino effecUve areas big neutrino telescopes 5 ) 10 TeV-PeV energy froner: 2 like KM3Net would 4 Km3Net, Baikal GVD 10 establish larger 3 detectors with more 10 2 sensiUvity from TeV to 10 PeV: 10 IC-80 up TL Astrophysical point 1 sources of neutrinos. -1 OpUmal view to GalacUc 10 -2 Antares 2007-8 Center (Southern 10 Hemisphere) Effective Neutrino Area (m -3 10 -4 10 IC-79 all sky PS -5 10 -6 10 1 2 3 4 5 6 7 8 9 10 11 log (Primary Neutrino Energy - GeV) Low energy extensions 10 to IceCube, DeepCore: PINGU, MICA 9 Water/ice Cherenkov detectors: Neutrino effecUve areas 5 ) 10 TeV-PeV energy froner: 2 UHE energy detectors 4 Km3Net, Baikal GVD 10 for GZK neutrino flux: 10 3 ARA, ARIANNA 10 2 10 IC-80 up TL Radio detecDon in ice: 1 Detecon volume and -1 thus sensivity 10 -2 Antares 2007-8 increases roughly 10 Effective Neutrino Area (m linearly with energy! -3 10 -4 10 IC-79 all sky PS -5 10 -6 10 1 2 3 4 5 6 7 8 9 10 11 log (Primary Neutrino Energy - GeV) Low energy extensions 10 to IceCube, DeepCore: PINGU, MICA 10 Low energies, 1 – 100 GeV, PINGU ... The Neutrino Detector Spectrum NOνA OPERA Accelerator based K2K, MINOS T2K Atmosphericl 10 MeV 100 MeV 1 GeV 10 GeV 100 GeV 1 TeV 10 TeV 1 EeV Solar/ Astrophysical Reactor Atmospheric IceCube/ANTARES ANITA/RICE/Auger/ KM3Net/baikal GVD ARA/ARIANNA borexino/KamLand/Daya Bay/Double Chooz/SNO/SuperK Non-accelerator based Slide: Courtesy Darren Grant NNN 2011 * boxes select primary detector physics energy regimes and are not absolute limits 11 Low energies, 1 – 100 GeV, PINGU ... The Neutrino Detector Spectrum NOνA OPERA Accelerator based K2K, MINOS T2K Atmosphericl 10 MeV 100 MeV 1 GeV 10 GeV 100 GeV 1 TeV 10 TeV 1 EeV Solar/ Astrophysical Reactor Atmospheric Gap IceCube/ANTARES ANITA/RICE/Auger/ KM3Net/baikal GVD ARA/ARIANNA borexino/KamLand/Daya Bay/Double Chooz/SNO/SuperK Dark Non-accelerator based Maer ντ Appearance νμ Neutrino Disappearance Mass Hierarchy Slide: Courtesy Darren Grant NNN 2011 * boxes select primary detector physics energy regimes and are not absolute limits 12 Low energies, 1 – 100 GeV, PINGU ... The Neutrino Detector Spectrum NOνA OPERA Accelerator based K2K, MINOS T2K Atmosphericl 10 MeV 100 MeV 1 GeV 10 GeV 100 GeV 1 TeV 10 TeV 1 EeV Solar/ DeepCore Astrophysical Reactor Atmospheric IceCube/ANTARES ANITA/RICE/Auger/ borexino/KamLand/Daya Bay/Double KM3Net/baikal GVD ARA/ARIANNA Chooz/SNO/SuperK PINGU Non-accelerator based beyond PINGU ~70 active members in feasibility studies: IceCube, KM3Net, Several neutrino experiments Photon detector developers Slide aer: Darren Grant Theorists NNN 2011 * boxes select primary detector physics energy regimes and are not absolute limits 13 Low energies, 1 – 100 GeV, PINGU ... IceCube-DeepCore • IceCube extended its “low” energy response with a densely instrumented infill array: DeepCore http://arxiv.org/abs/1109.6096" • Significant improvement in capabilities from ~10 GeV to ~300 GeV (νμ) • Scientific Motivations: • Indirect search for dark matter • Neutrino oscillations (e.g., ντ appearance) • Neutrino point sources in the southern hemisphere (e.g., galactic center) 14 Low energies, 1 – 100 GeV, PINGU ... IceCube - DeepCore: DESIGN http://arxiv.org/abs/1109.6096" •! Eight special strings in filled in the bottom center of IceCube •! ~5x higher effective photocathode density than regular IceCube IceCube! •! Result: ~20 MTon detector with % extra% ~10 GeV threshold, will collect % veto cap! O(100k) physics quality atmospheric "/yr! VETO AMANDA! •! IceCube’s top and outer layers of strings m provide an active veto shield for DeepCore! Deep % Core! 350 •! Effective #-free depth much greater! •! Atm. #/" trigger ratio is ~106! 250 m •! Vetoing algorithms expected to reach well beyond 106 level of background rejection (Muon flux after veto comparable to Sudbury depth)! 15 Low energies, 1 – 100 GeV, PINGU ... PINGU •! Phased IceCube Next-Generaon PINGU geometry Upgrade (more compact version also studied) •! Add 20 strings with ~1000 opUcal modules inside the Deep Core region (~500PMT) •! Expected energy threshold near 1 GeV •! R&D opportunity for future developments 100 Numu NHits 20 String PINGU V2 PRELIMINARY IC86 SMT3 Triggered 80 No Noise 4m PINGU DOM DOM Spacing 60 Average Number of Hits 40 20 Courtesy J. Koskinen 0 0 10 20 30 40 50 Neutrino Energy (GeV) 16 Simulated event in DeepCore and PINGU! DeepCore Only! • 8 GeV up-going muon neutrino (physics only hits)! DeepCore + PINGU! • No. of PMTs fired:! ! !Deep Core: 11 ! ! !PINGU: 83 ! 17 Courtesy J. Koskinen Neutrino PINGU Physics hierarchy Mena, Mocioiu & Razzaque, Phys. Rev. D78, 093003 (2008) 2 (sin (2θ13)=0.1) • Probe lower mass WIMPs • Gain sensitivity to second oscillation peak/trough • enhanced sensitivity to neutrino mass hierarchy ντ appearance • Gain increased sensitivity to supernova neutrino bursts νµ disappearance • Extension of current search for coherent increase in singles rate across entire detector volume • Only 2±1 core collapse SN/century in Milky Way • need to reach out to our neighboring galaxies • Gain depends strongly on noise reduction via Deep Core data coincident photon detection (e.g., in neighbor Resconi et al. (Poster) DOMs) Ref. on Supernova detecDon: G. Sullivan’s talk - L. Demiroers, M. Ribordy, M. Salathe arXiv:1106.1937 - Posters by M. Voge, R. Bruin Mass hierarchy Figure and Analysis from: Akhmedov, Razzaque, Smirnov, arXiv: 1205.7071 See poster by E. Resconi et al. (IceCube and PINGU) • Expected significance for observed number of events for IH vs NH are shown in energy vs. zenith plot • If required energy and direcUonal resoluUon is achievable: high stasUcal significance +6 18 +3 14 0 GeV 10 Conclusion (Akhmedov et al.): -1 Significance “Our preliminary esBmates show that aDer 5 years of PINGU 20 6 operaBon the significance of the determinaBon of the hierarchy can -2 range from 3 to 11 (without taking into account parameter [-1,08] [-0.8,-0.5] [-0.5,0.0] degeneracy), depending on the accuracy of reconstrucon of neutrino cos (theta) energy and direcon.” Assumed above: Energy resoluon: 4 GeV, Angular resoluDon: 0.3 in cos(theta) Exposure: 10 Mt yr 19 Low energies, 1 – 100 GeV, PINGU ..
Details
-
File Typepdf
-
Upload Time-
-
Content LanguagesEnglish
-
Upload UserAnonymous/Not logged-in
-
File Pages54 Page
-
File Size-