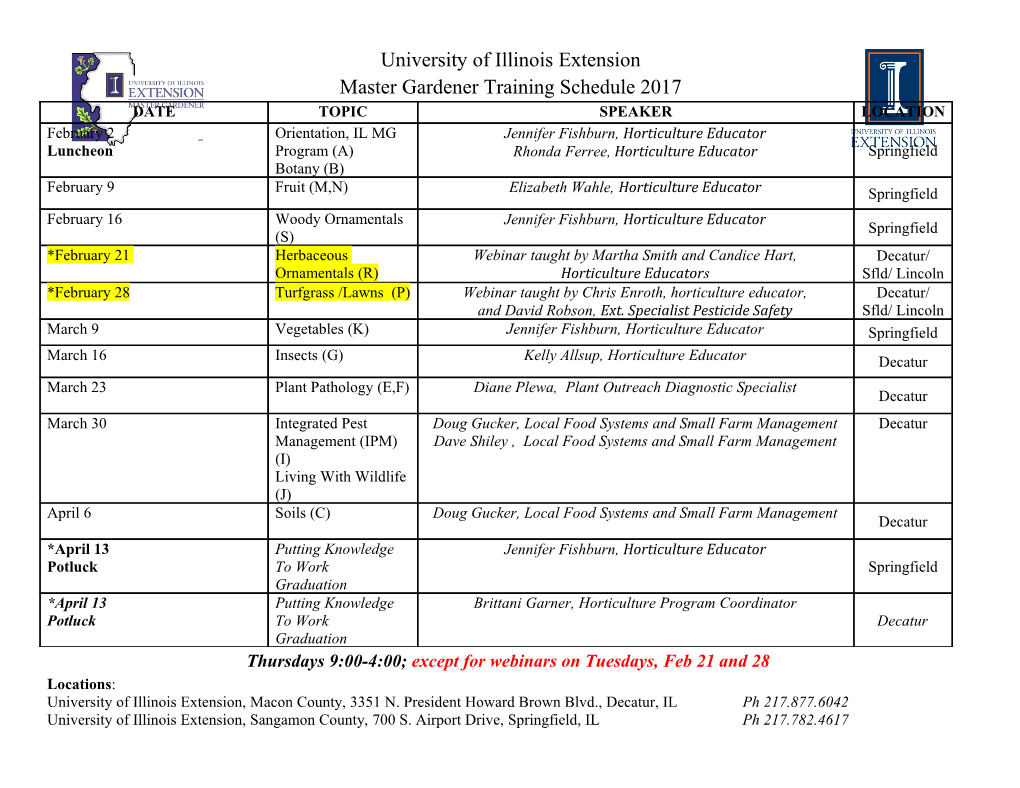
Single-crystal metal growth on amorphous insulating substrates Kai Zhanga,1, Xue Bai Pitnera,1, Rui Yanga, William D. Nixb,2, James D. Plummera, and Jonathan A. Fana,2 aDepartment of Electrical Engineering, Stanford University, Stanford, CA 94305; and bDepartment of Materials Science and Engineering, Stanford University, Stanford, CA 94305 Contributed by William D. Nix, December 1, 2017 (sent for review October 12, 2017; reviewed by Hanchen Huang, David J. Srolovitz, and Carl Thompson) Metal structures on insulators are essential components in advanced Our method is based on liquid phase epitaxy, in which the electronic and nanooptical systems. Their electronic and optical polycrystalline metal structures are encapsulated in an amor- properties are closely tied to their crystal quality, due to the strong phous insulating crucible, together with polycrystalline seed dependence of carrier transport and band structure on defects and structures of differing material, and heated to the liquid phase. grain boundaries. Here we report a method for creating patterned As the system cools, the metal solidifies into single crystals. single-crystal metal microstructures on amorphous insulating sub- Liquid phase epitaxy has been previously studied in the context of strates, using liquid phase epitaxy. In this process, the patterned semiconductor-on-oxide growth (24–26), but has not been ex- metal microstructures are encapsulated in an insulating crucible, plored for metal growth. We will examine gold as a model system together with a small seed of a differing material. The system is in this study. Gold is an essential material in electronics and heated to temperatures above the metal melting point, followed by plasmonics because of its high conductivity and chemical inertness. cooling and metal crystallization. During the heating process, the We use platinum as our seed material, which has a high melting metal and seed form a high-melting-point solid solution, which temperature and forms an isomorphous binary phase diagram with directs liquid epitaxial metal growth. High yield of single-crystal gold, with a miscibility gap at lower temperatures. metal with different sizes is confirmed with electron backscatter diffraction images, after removing the insulating crucible. Unex- Results and Discussion pectedly, the metal microstructures crystallize with the 〈111〉 direc- The device layout and fabrication flow are outlined in Fig. 1 A tion normal to the plane of the film. This platform technology will and B. To quantify crystal orientations and grain sizes in our enable the large-scale integration of high-performance plasmonic and electronic nanosystems. samples, we use electron backscatter diffraction (EBSD) imaging. Representative, large-area EBSD images of gold microstripes are C single-crystal metal | liquid phase epitaxy | solid-state diffusion | gold | presented in Fig. 1 , together with a scanning electron microscopy microcrucible (SEM) image of an individual stripe. The yield of single crystals for the 20 devices in the image is 100%. Interestingly, the orientations of the metal crystals are not random but have the h111i direction igh-quality metals are essential for obtaining desirable perpendicular to the substrate (i.e., along the z axis in the EBSD electronic and nanooptical properties in metal structures H images; see coordinates in Fig. 1C). In the directions along (x axis) and devices. For many of these properties, the elimination of and transverse (y axis) to the stripe, the crystal orientations are grain boundaries results in dramatic enhancements. In elec- random (SI Appendix,Fig.S1). Our process generally applies to a tronics, single-crystal metal structures have higher electrical conductivity and are more resistant to electromigration com- pared with polycrystalline structures (1–4). In plasmonics, single- Significance crystal metal devices possess exceptional plasmon propagation lengths (5) and electromagnetic field enhancements, which enable Eliminating grain boundaries of metal structures is desirable ENGINEERING high-performance waveguides (6, 7), sensors (8), antennas (9), for many electronic and nanooptical applications. However, a and metamaterials (10, 11). Metal crystal quality also plays a technique that can be used to grow large-scale patterned single-crystal metal on amorphous insulating substrate has not major role in catalysis (12, 13), photodetection (14, 15), energy been demonstrated. In this work, we show that using liquid harvesting (16, 17), and flexible electronics (18) due to the de- phase epitaxy, microstructures of single-crystal metal can be pendence of hot carrier dynamics, molecular kinetics, and me- grown on amorphous insulating substrates such as silicon di- chanical properties on defects and crystal orientation. oxide with high yield. The single-crystal metal can be further There currently exist two general routes to fabricating single- patterned on the insulating substrate with lithography into crystal metal structures on substrates. The first is to grow lattice- functional devices, which avoids the transfer, and is largely matched metal films onto crystalline substrates using molecular scalable. This technology platform will reduce the failure of beam epitaxy (19–21), electrodeposition, or physical vapor de- metal structures in electronic systems, and enable more effi- position (10, 11, 22) processes. These techniques are limited to the cient plasmonic nanostructures. use of either nonelectrically insulating or nonconventional substrate materials. The second is to chemically grow single-crystal metal Author contributions: K.Z., X.B.P., R.Y., J.D.P., and J.A.F. designed research; K.Z., X.B.P., colloids in solution (7–9, 23) and cast the structures onto a sub- R.Y., J.D.P., and J.A.F. performed research; K.Z., X.B.P., R.Y., W.D.N., J.D.P., and J.A.F. analyzed data; and K.Z., X.B.P., R.Y., W.D.N., J.D.P., and J.A.F. wrote the paper. strate, which cannot readily generalize to the construction of large- Reviewers: H.H., Northeastern University; D.J.S., University of Pennsylvania; and C.T., area integrated systems. To scientifically and technologically unlock Massachusetts Institute of Technology. the full potential of thin-film metal structures, we require new top- The authors declare no conflict of interest. down techniques that can produce single-crystal metal structures on Published under the PNAS license. amorphous insulating substrates, bypassing the requirement for di- 1K.Z. and X.B.P. contributed equally to this work. rect contact with single-crystal substrates, and with shapes and po- 2To whom correspondence may be addressed. Email: [email protected] or jonfan@ sitions patterned by lithography. Compatibility with substrate stanford.edu. materials such as silicon dioxide is critical for applications that re- This article contains supporting information online at www.pnas.org/lookup/suppl/doi:10. quire electrical isolation and a low refractive index background. 1073/pnas.1717882115/-/DCSupplemental. www.pnas.org/cgi/doi/10.1073/pnas.1717882115 PNAS | January 23, 2018 | vol. 115 | no. 4 | 685–689 Downloaded by guest on September 27, 2021 A Top view Side view C Pt y Au Si SiO2 substrate x z p-Au B SiO2 1s hold Si Grow 10800C crucible Anneal 001 Temp 150C/s 150C/s c-Au heating cooling Etch Time 101 111 crucible D z-axis E z-axis F z-axis y-axis y-axis y-axis x-axis x-axis x-axis Fig. 1. Liquid metal epitaxy process with gold microstripes and platinum seed structures. (A) Schematic of the structures before processing. (B) Schematic of the fabrication process flow. The images are cross-sectional views of the gold stripe away from the platinum seed region. (Inset) Diagram of the annealing parameters. (C) Large-area EBSD image of processed gold stripes. The color map (Lower Left) describes the orientations of the crystal plane normals. (Inset) SEM image of an individual gold stripe. The coordinate system denotes the axes for EBSD analysis: x axis along the stripe, y axis transverse to the stripe, and z axis normal to the stripe and substrate. (Scale bars: 20 μm.) (D) EBSD images of a representative processed gold stripe with platinum seed. (E) EBSD images of a representative gold stripe, as deposited by electron beam evaporation. (F) EBSD images of a representative gold stripe with no platinum seed, processed using the annealing parameters in B. (Scale bars for D–F:10μm.) broad range of microstructures, including stripes of tens to hun- platinum seed (Fig. 1F). This stripe is polycrystalline with ran- dreds of micrometers length and branch-like structures (SI Appen- domly oriented grains, indicating that upon system cooling, the dix,Figs.S2–S5). In devices that did not produce single crystals, we liquid gold crystallized with multiple random nucleation points observe microcrucible warping or failure, and we anticipate higher and crystal orientations. As such, the platinum seed is essential overall yield by using thicker and stiffer microcrucibles. to producing single-crystal gold structures. Detailed EBSD images of a representative processed gold A representative transmission electron microscopy (TEM) microstripe are presented in Fig. 1D and show that the seed image of a gold microstripe cross-section, with the top section of region, defined by the area of overlapping gold and platinum, is the oxide crucible etched away, is presented in Fig. 2. This 2.4-μm- polycrystalline with a h111i fiber texture. A closer examination of long film section possesses constant thickness and smooth the EBSD images reveals slight color variation of the gold sidewalls at the gold–silicon dioxide and gold–air interfaces. microstripe, indicative of some minor variations in orientation. Diffraction patterns from three different sections of the stripe all We also perform an EBSD analysis on two control samples. The show the same pattern and orientation, confirming that the first is of an as-deposited gold stripe without encapsulation or sample is an fcc single crystal. The nonuniform brightness within annealing (Fig. 1E). This stripe is polycrystalline with grains the diffraction spots is due to standard dynamical scattering ef- oriented with h111i directions normal to the plane of the film, fects.
Details
-
File Typepdf
-
Upload Time-
-
Content LanguagesEnglish
-
Upload UserAnonymous/Not logged-in
-
File Pages5 Page
-
File Size-