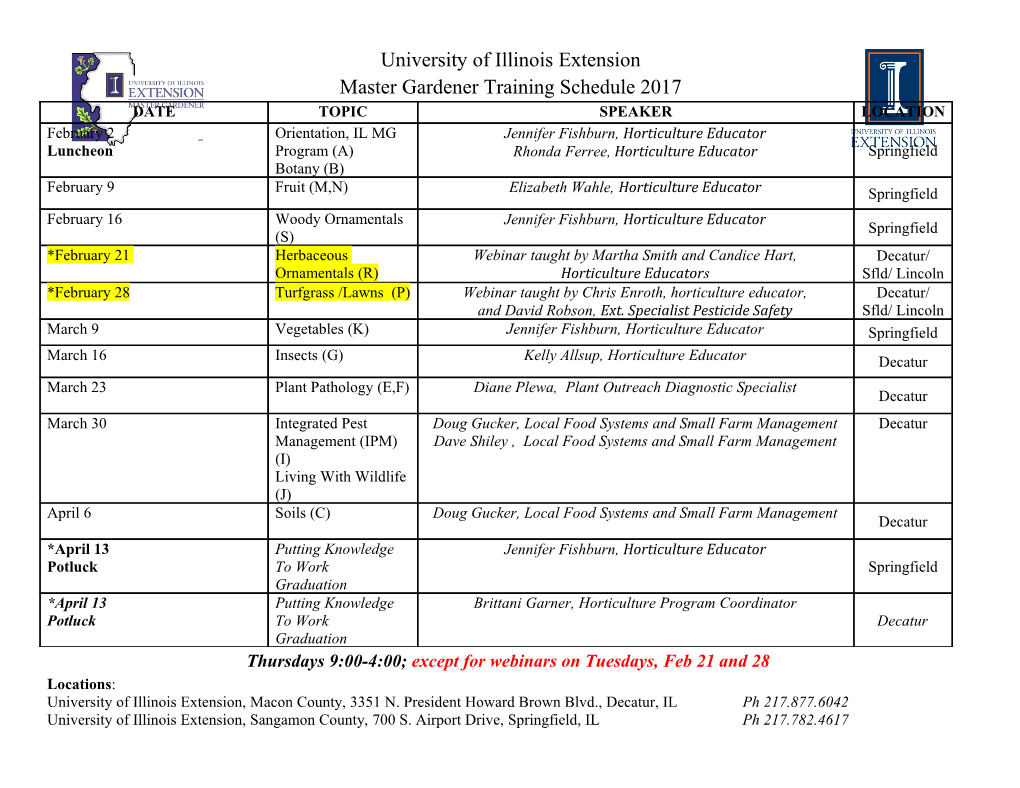
1SEPTEMBER 2014 H O M E Y E R E T A L . 6673 Assessing the Applicability of the Tropical Convective–Stratiform Paradigm in the Extratropics Using Radar Divergence Profiles CAMERON R. HOMEYER National Center for Atmospheric Research,* Boulder, Colorado COURTNEY SCHUMACHER Department of Atmospheric Sciences, Texas A&M University, College Station, Texas LARRY J. HOPPER JR. Department of Atmospheric Sciences, School of Sciences, University of Louisiana at Monroe, Monroe, Louisiana (Manuscript received 16 September 2013, in final form 15 May 2014) ABSTRACT Long-term radar observations from a subtropical location in southeastern Texas are used to examine the impact of storm systems with tropical or extratropical characteristics on the large-scale circulation. Clima- tological vertical profiles of the horizontal wind divergence are analyzed for four distinct storm classifications: cold frontal (CF), warm frontal (WF), deep convective upper-level disturbance (DC-ULD), and nondeep convective upper-level disturbances (NC-ULD). DC-ULD systems are characterized by weakly baroclinic or equivalent barotropic environments that are more tropical in nature, while the remaining classifications are representative of common midlatitude systems with varying degrees of baroclinicity. DC-ULD systems are shown to have the highest levels of nondivergence (LND) and implied diabatic heating maxima near 6 km, whereas the remaining baroclinic storm classifications have LND altitudes that are about 0.5–1 km lower. Analyses of climatological mean divergence profiles are also separated by rain regions that are primarily convective, stratiform, or indeterminate. Convective–stratiform separations reveal similar divergence char- acteristics to those observed in the tropics in previous studies, with higher altitudes of implied heating in stratiform rain regions, suggesting that the convective–stratiform paradigm outlined in previous studies is applicable in the midlatitudes. Divergence profiles that cannot be classified as primarily convective or stratiform are typically characterized by large regions of stratiform rain with areas of embedded convection of shallow to moderate extent (i.e., echo tops ,10 km). These indeterminate profiles illustrate that, despite not being very deep and accounting for a relatively small fraction of a given storm system, convection dominates the vertical divergence profile and implied heating in these cases. 1. Introduction vertical structure of heating play an important role in the large-scale circulation through the generation of poten- Atmospheric motions can occur, in part, because of tial vorticity anomalies. Houze (1982) observed that the the nonuniformity of diabatic heating processes associ- maximum heating (composed of latent, radiative, and ated with precipitating cloud systems. This concept is eddy sensible components) in stratiform rain regions oc- especially relevant in the tropics where variations in the curs at higher altitudes than in convective rain regions. Hartmann et al. (1984) used this observation to show that * The National Center for Atmospheric Research is sponsored the elevated heating profile associated with a mesoscale by the National Science Foundation. convective system (MCS) composed of both convective and stratiform precipitation regions was able to produce a much more realistic tropical dynamical response than Corresponding author address: Cameron Homeyer, National Center for Atmospheric Research, P.O. Box 3000, Boulder, CO a convective-only profile. Haynes and McIntyre (1987) 80305. and Mapes and Houze (1995) helped explain this result E-mail: [email protected] by showing that the generation of potential vorticity is DOI: 10.1175/JCLI-D-13-00561.1 Ó 2014 American Meteorological Society Unauthenticated | Downloaded 10/04/21 05:16 PM UTC 6674 JOURNAL OF CLIMATE VOLUME 27 directly proportional to the vertical gradient of the heat- more elevated divergence profiles. The fidelity of the ing profile in convective systems in the tropics. Many model simulations was dependent on the organization of studies since Hartmann et al. (1984) have examined how the system, which was in turn dependent on the baro- the vertical structure of tropical heating influences the clinicity of the environment. Model results were also dynamical response (e.g., DeMaria 1985; Sui and Lau sensitive to the microphysics and convective parame- 1989; Wu et al. 2000; Chiang et al. 2001; Schumacher et al. terizations and whether convection was parameterized 2004). on an intermediate 9-km nested grid. Both studies also Diabatic heating variations also play a role in the suggested that MCSs could have a significant feedback large-scale circulation in the midlatitudes, but their con- with the large-scale circulation at higher latitudes in tributions are not as well quantified or understood. Be- both warm-season and weakly baroclinic environments. ginning with Ninomiya (1971a), case studies have shown This study uses long-term radar observations in that midlatitude MCSs that cover large regions for ex- southeastern Texas, which experiences a diverse spec- tended periods or that occur in weakly baroclinic envi- trum of precipitating systems common in the tropics and ronments can have an active dynamic feedback with the midlatitudes, to evaluate the dynamical characteristics larger-scale circulation through vertical variations in dia- of storms based on their organization and synoptic batic heating, especially in the trailing stratiform rain re- forcing. This study’s overarching goal is to provide gion of an MCS (e.g., Hertenstein and Schubert 1991). a more quantitative context for assessing mesoscale– Many other midlatitude studies indicate that large con- synoptic interactions and the role precipitating sys- vective systems affect the local large-scale environment tems may have in large-scale flow outside of the by creating a large anticyclonic flow perturbation aloft, tropics. We do this by utilizing a multiyear subtropical intensifying upper-level divergence, and enhancing the dataset to observationally constrain the divergence large-scale baroclinicity (Ninomiya 1971b; Maddox et al. (and implied heating profiles) that may be used in fu- 1981; Raymond and Jiang 1990; Zhang and Harvey 1995; ture studies. The results have implications for the Stensrud 1996). Stensrud and Anderson (2001) further modeling and forecasting of a variety of extratrop- argued that regions of persistent midlatitude convection ical storms while also evaluating how applicable the can affect the hemispheric circulation and Chang et al. convective–stratiform paradigm (see Houze 1997)isat (2002) described how diabatic heating from condensa- higher latitudes. tional processes strengthens midlatitude storm tracks. In addition, horizontal variations in diabatic heating within frontal storm systems have been shown to strengthen 2. Methods frontogenesis (Bryan and Fritsch 2000; Wakimoto and a. Storm classifications Murphey 2008). However, very little research exists be- yond individual case studies on the relationship between Four classifications are used to categorize storms precipitating systems and the large-scale circulation out- depending on the primary mechanism responsible for side of the tropics. initiating convective or nonconvective precipitation. Hopper and Schumacher (2009, 2012) attempted to Surface and upper-air maps, satellite images, and sur- rectify this void by using a larger set of cases and a com- face radar reflectivity images from the online archive bination of mesoscale modeling and observations to an- maintained by the National Center for Atmospheric alyze microphysical and dynamical differences between Research (NCAR) Mesoscale and Microscale Meteo- storms of varying organization [e.g., leading-line trailing- rology Division (online at http://www.mmm.ucar.edu/ stratiform (LLTS) MCSs versus less organized systems] imagearchive/) have been matched with the indepen- occurring in a range of synoptic conditions (i.e., warm dent radar data used in this study and analyzed to season, weakly baroclinic, and strongly baroclinic) in determine each storm’s classification. Archives of me- southeastern Texas. Microphysical comparison metrics soscale discussions and mesoanalyses from the National included stratiform rain production and vertical profiles Weather Service Storm Prediction Center and vertical of reflectivity, while dynamical metrics included vertical cross sections of radar data are also used in classifying velocity and divergence profiles. Divergence is especially some storms. In this study, we consider that stratiform useful because it can be linked back to diabatic heating rain can originate from 1) deep, vertically oriented profiles (e.g., Mapes and Houze 1995) and is more easily convective sources (deep convection) and 2) synoptic- observable than vertical velocity and diabatic heating. scale lifting without a deep convective source that does Hopper and Schumacher (2009, 2012) found that storms not exclude slantwise, elevated, or shallow convection occurring in less baroclinic environments have more (nondeep convection). The composite schematics illus- convective rain area, less stratiform rain production, and trated in Fig. 1 depict representative cases for these Unauthenticated | Downloaded 10/04/21 05:16 PM UTC 1SEPTEMBER 2014 H O M E Y E R E T A L . 6675 FIG. 1. Composite figures of representative cases for (a) cold frontal, (b) warm frontal, (c) deep convective upper- level disturbance (ULD), and (d) nondeep convective ULD storm classifications. All images are adapted
Details
-
File Typepdf
-
Upload Time-
-
Content LanguagesEnglish
-
Upload UserAnonymous/Not logged-in
-
File Pages14 Page
-
File Size-