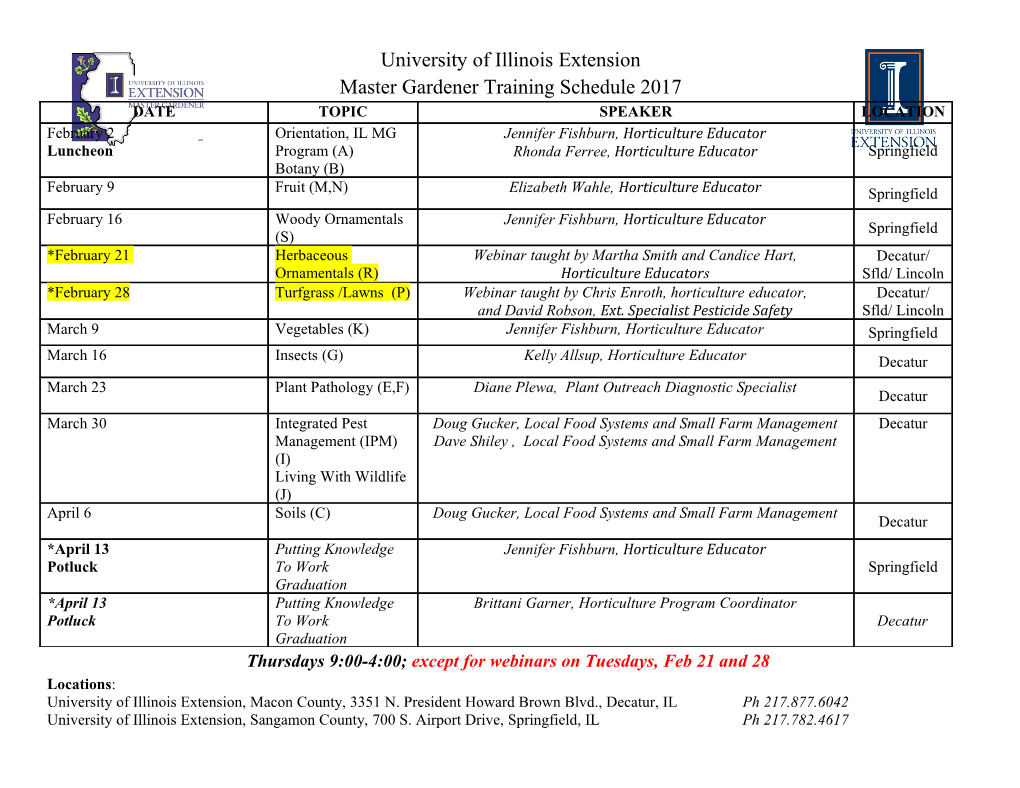
J Pharmacol Sci 96, 249 – 254 (2004) Journal of Pharmacological Sciences ©2004 The Japanese Pharmacological Society Forum Minireview Molecular Mechanisms and Drug Development in Aquaporin Water Channel Diseases: Molecular Mechanism of Water Channel Aquaporin-2 Trafficking Yumi Noda1,* and Sei Sasaki1 1Department of Nephrology, Graduate School, Tokyo Medical and Dental University, Tokyo 113-8519, Japan Received September 29, 2004; Accepted October 13, 2004 Abstract. Targeted positioning of water channel aquaporin-2 (AQP2) strictly regulates body water homeostasis. Trafficking of AQP2 to the apical membrane is critical for the reabsorption of water in renal collecting ducts. Besides the cAMP-mediated effect of vasopressin on AQP2 trafficking to the apical membrane, other signaling cascades also induce this sorting. Recently, AQP2-binding proteins that directly regulate this trafficking have been uncovered: SPA-1, a GTPase-activating protein (GAP) for Rap1, and cytoskeletal protein actin. This review summarizes recent advances related to the trafficking mechanism of AQP2 and its defect causing nephrogenic diabetes insipidus (NDI). Keywords: aquaporin-2, PKA phosphorylation, missorting, Rho, cytoskeleton Introduction receptors located in the hypothalamus are activated and stimulate the secretion of antidiuretic hormone arginine Body water homeostasis is essential for survival of vasopressin (AVP) from the posterior pituitary (22, 23). mammals. Water transport occurs through a specialized After it is released systemically, vasopressin binds to channel called aquaporin (AQP) (1 – 4). AQPs play an vasopressin type 2 receptor (V2R) located on the baso- important role in reabsorption of water and in concentra- lateral membrane of the renal collecting duct principal tion of urine in the kidney. Out of 13 aquaporin iso- cells (24). The V2R is coupled to adenylate cyclase by forms, AQP2 is the predominant vasopressin-regulated the heterotrimeric G-protein, Gs. Gs is a guanosine water channel (5, 6). The vasopressin tightly regulates triphosphate (GTP)-binding protein that consists of three body water balance. Upon vasopressin stimulation, subunits: , , and . The binding of vasopressin to its AQP2 translocates from subapical storage vesicles to receptor causes the -subunit to release guanosine the apical plasma membrane, rendering the cell water diphosphate (GDP), take up intracellular GTP, and permeable, which in turn causes water reabsorption dissociate from the - and -subunits. This G -GTP leading to urine concentration. AQP2 mutations cause complex, in turn, activates adenylate cyclase which congenital nephrogenic diabetes insipidus (NDI), a catalyzes the conversion of ATP to cyclic adenosine disease characterized by a massive loss of water through monophosphate (cAMP). The subsequent activation of the kidney (7 – 21). Proper trafficking of AQP2 is protein kinase A (PKA) leads to phosphorylation of critically important for body water homeostasis. In this AQP2 at serine 256 (25). This phosphorylation event is review we focus on the trafficking mechanism of AQP2. required for AQP2 translocation from subapical storage vesicles to the apical membrane which causes an AQP2 trafficking mediated by PKA phosphorylation increase in water permeability of renal principal cells and other pathways (25 – 28). The increase in permeability allows water to flow from the tubule lumen to the hypertonic medullary In response to an increase in serum osmolarity and a interstitium. This then leads to the formation of concen- reduction in effective circulating blood volume, osmo- trated urine. Withdrawal of vasopressin triggers the endocytosis of AQP2-containing vesicles and restores *Corresponding author. FAX: +81-3-5803-5215 the water-impermeable state of the apical membrane. E-mail: [email protected] 249 250 Y Noda and S Sasaki AQP2 is expressed as a homotetramer, and for plasma state of this water channel at serine 256 (46). Phosphory- membrane localization, at least three out of four mono- lation of other cytoplasmic or vesicular regulatory mers in an AQP2 tetramer have to be phosphorylated proteins may also be involved. These issues remain to (18, 29). be investigated directly. As PKA and many of its substrates are present Other alternative (or parallel) trafficking pathways throughout the cell, localization of PKA to specific sites are also being uncovered. Bouley et al. have shown that is necessary for a timely and spatially effective acti- nitric oxide and atrial natriuretic factor stimulate the vation of PKA. This process is mediated by protein insertion of AQP2 in renal epithelial cells via a cGMP- kinase A anchoring proteins (AKAPs) that tether PKA to dependent pathway (47). It is not yet clear whether specific sites and limit its access to a subset of substrates PKG directly phosphorylates AQP2 or whether the (30 – 32). Lande et al. found that AQP2-bearing vesicles cGMP/PKG effect is ultimately mediated by activation contained PKA activity (33). The anchoring of PKA to of PKA. AKAPs was demonstrated to be a prerequisite for the AQP2 shuttle using the membrane-permeable anchoring Role of the cytoskeleton inhibitor peptide S-Ht31 (34 – 36). Henn et al. found that a new splice variant of AKAP18, AKAP18, was The coordinated delivery of AQP2-bearing vesicles involved in this AQP2 shuttle (37). appears to depend on the translocation of the vesicles However, the role of AQP-2 phosphorylation in along the cytoskeletal elements. The microtubular AQP-2 trafficking is unclear. Recent work has demon- network has been implicated in this process (48 – 50). strated that the serine/threonine phosphatase inhibitor Microtubules are polar structures, arising from micro- okadaic acid induces AQP-2 translocation even in the tubule organizing centers (MTOCs), at which their presence of H89, a specific PKA inhibitor (38). Thus, minus ends are anchored, and with the plus ends grow- AQP-2 translocation may involve a mechanism that is ing into the cell. Dynein is shown to be present in the independent of PKA phosphorylation in renal collecting- kidney, and both dynein and dynactin, a protein complex duct cells. believed to mediate the interaction of dynein with Several studies have provided evidences for a role of vesicles, associate with AQP2-bearing vesicles (51). ryanodine-sensitive Ca2+ stores and calmodulin in Both vanadate, an inhibitor of ATPases, and erythro-9- vasopressin-mediated AQP-2 trafficking. In addition to (2-hydroxy-3-nonyl) adenine (EHNA), an inhibitor of increasing cAMP levels in collecting duct principal dynein, inhibit the antidiuretic response in toad bladder cells, vasopressin binding to the V2 receptor was also (52, 53). Thus, the microtubule-associated motor protein demonstrated to transiently increase intracellular Ca2+ dynein and dynactin are important in vasopressin-regu- (39 – 42). Both ryanodine and calmodulin inhibitors lated trafficking of these vesicles. were shown to block vasopressin-stimulated transloca- A reorganization of the actin cytoskeleton has also tion of AQP-2 to the plasma membrane (43, 44). Thus, been shown to be essential in the trafficking of AQP2- vasopressin-induced intracellular calcium release from containing vesicles. It has been suggested that the actin ryanodine-sensitive stores plays a critical role in the cytoskeleton provides a network that anchors the translocation of AQP2 to the apical membrane. AQP2-bearing vesicles in the unstimulated cell and that While PKA phosphorylation is required for the vaso- a reorganization of the apical actin network may be pressin-induced cell-surface accumulation of AQP2, critical in promoting the trafficking of AQP2-bearing dephosphorylation of AQP2 is probably not necessary vesicles. Indeed, vasopressin has been shown to depoly- for its subsequent internalization. Prostaglandin E2 stim- merize apical F-actin in rat inner medullary collecting ulates removal of AQP2 from the surface of principal duct, resulting in the fusion of water channel-carrying cells when added after vasopressin treatment but does vesicles with the apical membrane (54). Both forskolin not alter the phosphorylated state of AQP2 (28). and okadaic acid stimulate AQP-2 translocation by Phosphorylation of AQP2 by other kinases may inducing a reorganization of the apical actin network potentially participate in regulation of AQP2 trafficking. (38). Recent studies showed that actin depolymerization Besides PKA sites, putative phosphorylation sites for was a prerequisite for cAMP-dependent translocation of PKG, PKC, and casein kinase II are also present in the AQP2 and inhibition of small GTPase Rho induced AQP2 sequence. Ser-256 is also a substrate for Golgi this translocation by causing actin depolymerization casein kinase and its phosphorylation by this kinase is (55, 56). These data provide strong evidence for a major required for Golgi transition (45). It was shown that regulatory role of the actin depolymerization in the activation of the PKC pathway mediated endocytosis of vasopressin-induced recycling of AQP2 between intra- AQP2, which was independent of the phosphorylation cellular vesicles and the cell surface. Furthermore, Trafficking of Water Channel Aquaporin-2 251 RhoA inhibition through PKA-mediated phosphoryla- mediated endocytosis (74 – 76). Phosphorylation-defi- tion of RhoA is shown to be a key event for cytoskeletal cient AQP2 mutant S256A accumulates at the plasma dynamics inducing AQP2 translocation (57). In addition, membrane by the GTPase-deficient dynamin mutant Tamma et al. showed
Details
-
File Typepdf
-
Upload Time-
-
Content LanguagesEnglish
-
Upload UserAnonymous/Not logged-in
-
File Pages6 Page
-
File Size-