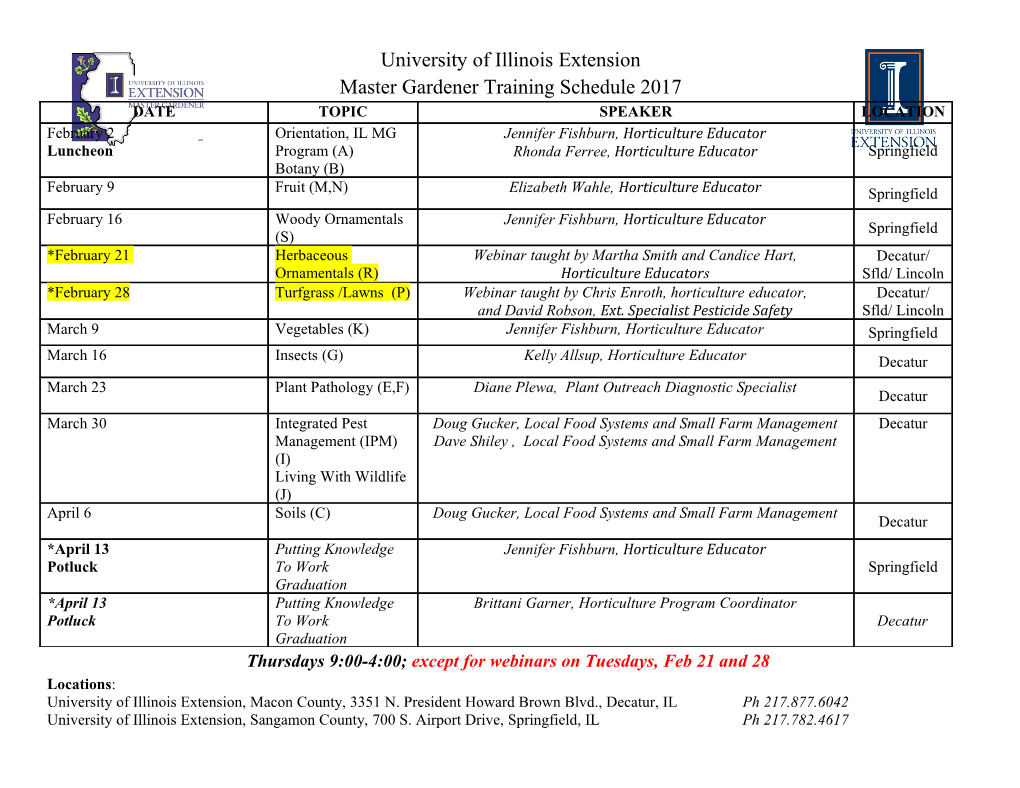
Electric propulsion for Mars cargo missions ASEN 5053 Final Project Amal Chandran May 5, 2007 1 Contents 1. Introduction……………………………………..3 2. Mission Scenario………………………………..4 3. cargo mission mass requirements…………….....5 4. Cargo mission Launcher………………………...6 5. Electric propulsion……………………………....7 6. Solar Electric propulsion………………………..8 7. Nuclear electric propulsion…………………….10 8. Emerging Technologies……………………..…13 9. Conclusion……………………………………..14 10. References……………………………………..15 List of Figures Fig 1: Mars Cargo Mission [1]………………………………………………….…4 Fig 2: Mars mission stages 3, 4, and 5 [1] ……………………………….…….….5 Fig 3: ARES V launch image Courtesy NASA……………………………..….….6 Fig 4: Electric propulsion systems[2] ……………………………………….….…7 Fig 5:Variation in initial mass in LEO with trip time for different Isp[3] ….….….8 Fig 6: Schematic of SEP cargo vehicle[3] ………………………………………...9 Fig 7: Mass break down for SEP Mars cargo vehicle[3] ……………………….…9 Fig 8: NEXIS 57 cm diameter ion thruster[5] …………………………………....10 Fig 9: Schematic of a NEP cargo vehicle[6] ……………………………………..12 Fig 10. Conceptual design of ALFA2 Thruster[5] ………………………………..13 Fig 11: A Mars Exploration Camp courtesy www.marsproject.com…………..14 List of Tables Table 1: Mass breakdown for Chemical Rocket………………………………….5 Table 2: Mass breakdown for Nuclear thermal Propulsion……………………….6 Table 3: Mass breakdown for conceptual NEP vehicle………………………….12 Table 4 : CaLiPPSo and ALFA2 comparison [5] ………………………………...13 2 1. Introduction The human exploration of Mars is felt by many to be long overdue. When the first humans walked on the surface of the moon, the exploration of Mars was expected to follow inevitably, but this did not happen. Nevertheless, there remains a strong feeling that a human expedition to Mars will happen someday. One of the major obstacle involved in a human exploration mission to Mars is the huge payloads that have to be transported from Earth and inserted into a Martian orbit. This will require a huge amount of propellant and drive up the cost. A solution to this would be to ferry the propellant for the return journey as well as Martian exploration vehicles in advance on a unmanned cargo space craft into Martian orbit and then later let the piloted crew vehicle rendezvous with it in Martian orbit. Electric propulsion offers a cost effective and promising alternative to conventional propulsion because of the impulses it generates. A major drawback with Electric propulsion though is the low thrust it generates. In electric propulsion, electric energy from solar cells or nuclear-electric reactor is used to energize the propellant working fluid to yield specific impulses much higher than those available from chemical reactions.[2] This reduces the propellant requirement for a given spacecraft ∆V. Electric propulsion devise are “power limited” since the rate at which energy from the external source is supplied to the propellant is proportional to the mass of the power system. This results in very low thrust levels for a given vehicle mass. Hence electric propulsion vehicles are low acceleration vehicles with low thrust to weight (T/W) ratio. However Electric propulsion systems are ideal for non time critical interplanetary trajectories like cargo transfer missions. This Research paper analyses the different Electric propulsion techniques and takes an in depth look at a few Mars cargo ferries using electric propulsion that have been proposed in the past. 3 2. Mission Scenario First stage – A cargo transport carrying the landing vehicle and the earth return propellant, which has been assembled in LEO (500 km for this project) will be launched via an expendable vehicle on a minimum energy trajectory. Second stage- Upon arrival at Mars this vehicle will be placed in Low Mars Orbit (6000 km for this project) to await piloted human crew vehicle. An orbit of 6000 km is the same as that of Mar’s inner moon Phobos. The cargo vehicle could be landed on Phobos and power from the Electric propulsion devices could be used to extract resources such as water from Phobos for production of propellant or other useful materials. Third stage – Approximately 15 months after the first launch a vehicle carrying the crew members will be launched on a high energy low duration trajectory called a ‘sprint’ trajectory Fourth stage – Upon arrival at Mars the piloted vehicle will rendezvous with the cargo vehicle and depart for exploration of the Martian surface. The crew members remaining in the crew vehicle will transfer the propellant and other cargo from the cargo vehicle to piloted vehicle to prepare for the return journey to Earth. Fifth Stage – The surface crew will rendezvous with the orbiting piloted vehicle to depart for Earth, arriving about 5 months later. Figure 1 illustrates the mission scenario. Fig 1: Mars Cargo Mission [1] 4 Figure 2 illustrates Mars Orbit Capture, piloted crew vehicle docking with the cargo, lander going to Martian surface. Ascent vehicle returning after exploration and finally the crew vehicle returning to Earth. Figure 2: Mars mission stages 3, 4, and 5 [1] 3. Cargo Mission Mass requirements Earlier designs proposed a chemical rocket as the piloted crew vehicle which requires huge amount of propellants of the order of ~ 400,000kg Table 1: Mass breakdown for Chemical Rocket Payload Type Weights (kg) Earth return propellant 400,000 ERV 65,000 Mars excursion module (Lander) 55,000 Aeroshell 10,000 Propellant 50,000 Stage Dry mass 25,000 Total Mass 605,000 5 Using a Nucler thermal Propulsion for piloted vehicle results in huge mass savings.For purposes of this project 65 MT payload derived from NASA Human Exploration of Mars Design Reference Mission (DRM) version 3.0 has been used[3].. This payload corresponds to delivery of an Earth Return Vehicle (ERV) into Mars orbit. In the nominal DRM 3.0 A Nuclear thermal propulsion stage is used for Earth escape and trans-Mars Injection (TMI). The NTP stage is then jettisoned and the payload is captured into orbit. Here we assume this function is performed by the cargo transport vehicle [3]. Table 2: Mass breakdown for Nuclear thermal Propulsion Payload Type Weight (kg) ERV 65,000 Mars excursion module (Lander) 55,000 Aeroshell 10,000 Propellant 50,000 Stage Dry mass 25,000 Total Mass 205,000 This payload can be brought in to Mars orbit by a single cargo vehicle or in two cargo ferries [3] and assembled in Mars orbit. One of which has the ERV as the main payload and the other has the Mars excursion module as the primary module an additional propellant mass of 45,000 and stage dry mass of 25,000 will be added in this case, with one vehicle weighing ~140,000 kg (ERV) and the other (ML) weighing ~ 135,000 kg 4. Cargo mission launcher for ETO The Ares V launch vehicle which has a payload capacity of 125,000 kg to LEO is chosen as the launch vehicle. Figure 3: illustrates an ARES V launch. For a Chemical rocket Earth Return Vehicle 6 launches of ARES V followed by in-space assembly is required for assembling the cargo ferry in LEO. For a Nuclear Thermal Propulsion ERV, Each Cargo ferry will have masses of the order of 130 tonnes to 140 tonnes. Each will require two launches each of the ARES V launch vehicle followed by assembly in LEO. Figure 3: ARES V launch image Courtesy NASA 6 5. Electric Propulsion In electric propulsion, electric energy from solar cells or nuclear-electric reactor is used to energize the propellant working fluid to yield specific impulses much higher than those available from chemical reactions.[2] This reduces the propellant requirement for a given spacecraft ∆V. Electric propulsion devise are “power limited” since the rate at which energy from the external source is supplied to the propellant is proportional to the mass of the power system. This results in very low thrust levels for a given vehicle mass. Hence electric propulsion vehicles are low acceleration vehicles with low thrust to weight (T/W) ratio. Electric propulsion systems are therefore ideal for non time critical interplanetary trajectories like cargo transfer missions. Fig 4 illustrates an electric propulsion system. An Electric Propulsion system consists of a power (solar or nuclear) system power conditioning, thruster and propellant storage and feed subsystem. Fig 4: Electric propulsion systems[2] The Mars cargo mission is to be designed for transporting payload from LEO to a 6000 km altitude Low Mars Orbit (LMO). Typical ∆Vs for low T/W LEO to LMO transfers are of the order of 16 km/s[3]. As mentioned earlier, this LMO is at the same altitude as the inner moon, Phobos. An Earth to Mars trip time of 2.2 years to match the Earth-Mars synodic period is chosen. This makes it possible to launch the cargo vehicles during one Trans-Mars Injection (TMI) opportunity, travel to Mars, perform Mars orbit insertion and check out all the payload systems prior to launching the crew during the next mars TMI. The power requirement for these missions are of the order of 1000 – 2000 KW or 1- 2 MW as can be seen from figure 3. So, a one way expendable megawatt-class cargo vehicle is required. For this project an Ion (Herakles) thruster which was proposed for the Jupiter Icy Moons Orbiter (JIMO) mission and is considered a near term advanced thruster, is assumed to be used. Figure 5 plots the Initial mass in LEO against Earth–Mars trip time. It compares the Xe-Herakles thruster at different Isp’s of 6000, 7000 and 8000 7 seconds with the Bi-VHITAL (Very High Isp Thruster with Anode Layers) thruster at Isp’s of 5000, 6000 and 7000 seconds[3]. Fig 5:Variation in initial mass in LEO with trip time for different Isp[3] Power systems for electric propulsion can be either solar or nuclear power based.
Details
-
File Typepdf
-
Upload Time-
-
Content LanguagesEnglish
-
Upload UserAnonymous/Not logged-in
-
File Pages15 Page
-
File Size-