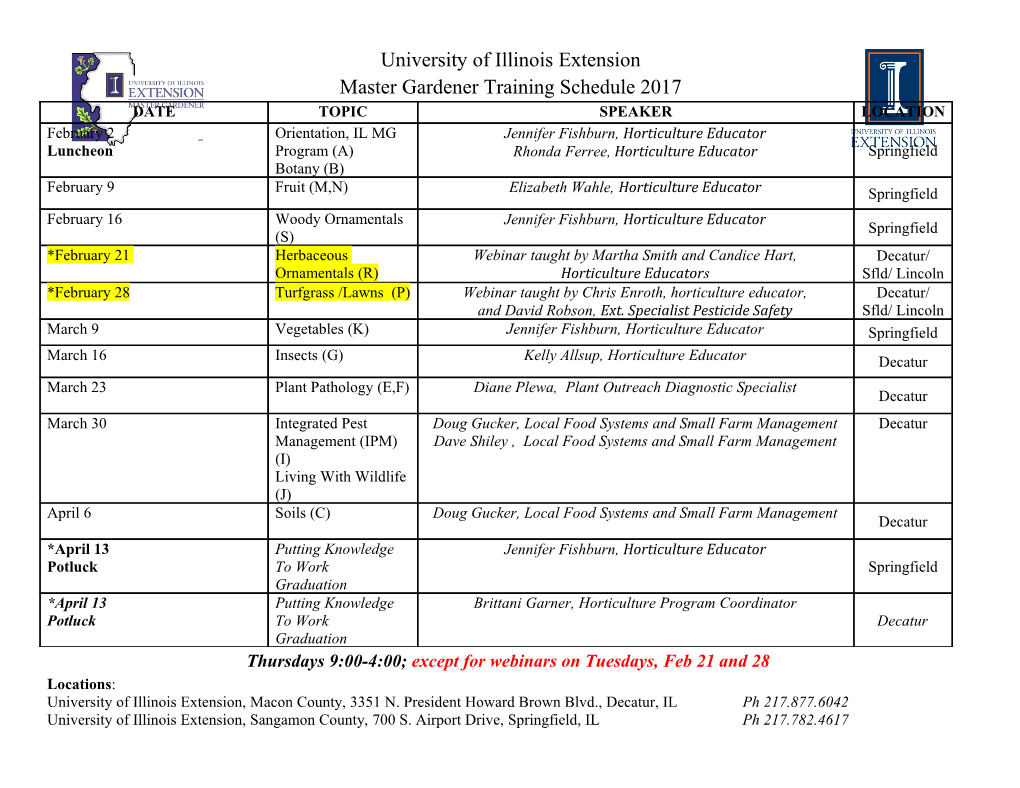
Characterisation of the MICE Experiment Sophie Charlotte Middleton High Energy Physics Blackett Laboratory Imperial College London A Thesis submitted in partial fulfilment of the requirements of the degree of Doctor of Philosophy in the Department of Physics at Imperial College London August 8, 2018 Abstract Muon beams of low emittance would deliver intense, well-characterised neutrino beams necessary to explicate the physics of flavour at a Neutrino Factory and for high-luminosity lepton-antilepton collisions at a multi- TeV muon collider. The International Muon Ionisation Cooling Experiment (MICE), based at the Rutherford Appleton Laboratory, aims to demonstrate ionisation cooling, the technique proposed to reduce the emittance of muon beams at such facilities. An ionisation cooling channel has been constructed. The muon beam traverses a low-Z absorber material, losing energy via ionisation. The phase space volume occupied by the beam is reduced, resulting in transverse cooling. This thesis presents two independent analyses accomplished through exploiting data obtained during the \Step IV" commissioning of MICE. Muons can decay within the cooling channel. The presence of electron contaminants within MICE will generate systematic uncertainties on the cooling measurement. The angular distribution of decay electrons is dependent upon the muon polarisation. It is, therefore, imperative to characterise the impact of depolariza- tion in the channel. Chapter 4 presents a unique measurement of the polarisation of the MICE muon beam at the downstream calorimeter. For an initially unpolarised muon beam a polarisation of: -0.021 ± 0.243 (stat.) ± 0.185 (sys.) ± 0.007 (depol.) ± 0.001 (det.) is obtained, appropriately identifying the polarisation of the beam at the point of decay, within acknowledged errors. MICE is devised to possess an on-axis magnetic field. It is paramount that misalignment in the cooling channel is characterised. Chapter 5 ascertains a measurement of the central Focus Coil's transverse position using single particle transfer matrices. No misalignment of the Focus Coil's magnetic axis, relative to the beam axis, is observed, within the limits of the analysis. This innovative technique can be employed by any multi-element accelerator system where particle co-ordinates are quantified upon entering and exiting a constituent magnet. Declaration of Originality The work presented in this thesis is my own unless explicitly stated otherwise. Chapters 1 and 2 introduce and expatiate upon accepted and verified concepts of contemporary particle and accelerator physics respectively. Discussion of such is necessary to present a comprehensive overview of the motivations for construction of the Muon Ionisation Cooling Experiment. All major results are referenced where appropriate. Chapter 3 gives a synopsis of the design goals of the Muon Ionisation Cooling Experiment together with a detailed discussion of the various hardware and software techniques employed by the experiment in order to accomplish its aims. Chapter 4 and 5 detail two independent analyses. The analysis techniques outlined in these chapters are entirely my own work, from conception to implementation, unless explicitly stated. The analysis pro- cedures outlined in both chapters were novel and unique. The method exhibited in Chapter 5 provides an innovative approach to calculating magnet misalignment using the transfer matrices of single particles. A measurement of this kind has never been done before. Both analyses exploit existing libraries and data structures inherent within the MICE Analysis User Software (MAUS). However, in order to complete the polarisation study, presented in Chapter 4, substantial upgrades to MAUS were carried out by myself. These gave MAUS the functionality which permitted the mon- itoring of particle spin and utilised the GEANT4's spin tracking algorithms. The data used in both Chapters 4 and 5 belongs to the MICE experiment the data is structured according to the standardised MAUS libraries. It is noted in Chapter 2 that MICE must gain a better understanding of Multiple Coloumb Scatter- ing which acts to counter ionisation cooling. Throughout the first year of my PhD I carried out a preliminary study which compared numerous models of multiple scattering and energy loss to data from the MuScat experiment, a predecessor to MICE. Chapter 2 presents some of the results of that study and utilises data obtained by MuScat. A primitive version of the MAUS simulation is compared to the data along with ICOOL and various versions of GEANT4. As part of this study I made some upgrades to MAUS which aimed to characterise the processes of multiple scattering and energy loss with the intention being to acquire data and build improved, data-based, models of both physical processes for the MICE absorber materials. However, due to insufficient MICE data at the time this work was discontinued at the beginning of 2014 and will now be completed by other members of the collaboration. There was no data from MICE available to compare with simulation at the point this thesis was originally written and therefore this discussion is only included to help illustrate the need for further study. -Sophie Charlotte Middleton 1 Copyright Declaration The copyright of this thesis rests with the author and is made available under a Creative Commons Attribution Non-Commercial No Derivatives licence. Researchers are free to copy, distribute or transmit the thesis on the condition that they attribute it, that they do not use it for commercial purposes and that they do not alter, transform or build upon it. For any reuse or redistribution, researchers must make clear to others the licence terms of this work. -Sophie Charlotte Middleton 2 Acknowledgments This thesis is dedicated to my Mum, Bernadette, for her unwavering support throughout my academic life and for providing me with the confidence that made my dream a reality. Everything I have achieved is due to your encouragement, Thank You. I'd like to express my gratitude to David Colling for allowing me the opportunity to pursue a PhD in the High Energy Physics group. It is an one I am very grateful to have had. Additional thanks must be granted to my colleagues, both at Imperial and on the MICE experiment, for making this rollercoaster such an enjoyable ride. It has been a pleasure to work amongst such intelligent and friendly people. In particular I'd like to show my appreciation to both Melissa and Celeste for all the time we spent drinking, chatting and even singing into the early morning after long days of collaboration meetings and additionally for your support over the final year. My final acknowledgement goes to my sister, Phoebe, and to all the friends I've made during my time in London for always being there at the end of a hard day for a good night out - thanks for the memories and for making my time in this absurd city the best time of my life. \We look for stranger things, because that's just who we are." |Kyrre Gørvell-Dahll 3 Contents 1 Introduction & Motivations 1 1.1 The Standard Model . 1 1.1.1 Overview: Fundamental Particles . 1 1.1.2 Phenomenology of the Standard Model . 3 1.1.3 Spontaneous Symmetry Breaking and The Higgs Mechanism . 5 1.1.4 Understanding The Higgs Boson . 6 1.2 Beyond the Standard Model . 6 1.3 Multi-TeV Muon Colliders . 9 1.3.1 The Large Hadron Collider and HL-LHC . 10 1.3.2 Electron-Positron Linear Colliders . 10 1.3.3 Muon Colliders . 10 1.3.4 Choosing Between a Muon Collider and e+e− Linear Collider . 14 1.4 The Impact of Neutrino Mass . 14 1.4.1 Phenomenology of Neutrino Oscillations . 14 1.4.2 Matter Effects on Neutrino Oscillations . 17 1.4.3 The Neutrino Mass Hierarchy . 17 1.4.4 CP Violation and Measuring δ ............................... 19 1.4.5 Nature of Neutrinos - Dirac or Majorana? . 20 1.4.6 Neutrino Helicity - The Existence of Sterile Neutrinos? . 20 1.4.7 Current Experimental Limits . 21 1.4.8 Future Experiments . 22 1.5 The Neutrino Factory . 23 1.6 Summary . 25 2 Ionisation Cooling 26 2.1 Co-ordinate Systems . 26 2.1.1 Phase Space . 26 2.1.2 Trace Space . 27 4 2.2 Single Particle Motion: Transfer Maps . 27 2.2.1 The Hamiltonian for Particle Motion in Accelerators . 27 2.2.2 First Order Transfer Maps . 29 2.2.3 Higher Order Transfer Maps . 31 2.2.4 Properties of the First Order Transfer Map . 32 2.3 Collective Motion and Beam Emittance . 33 2.3.1 Liouville's Theorem . 33 2.3.2 The Covariance Matrix . 34 2.3.3 Geometric Interpretation of Single Particle Emittance . 35 2.3.4 Defining Beam Emittance . 36 2.3.5 Normalised Beam Emittance . 37 2.3.6 Normalised Beam Emittance in MICE . 37 2.3.7 Angular Momentum in Solenoidal Optics . 38 2.3.8 Covariance Matrix in 4D Solenoid Optics . 38 2.3.9 Acceptance . 39 2.4 Beam Cooling . 39 2.4.1 Traditional Cooling Techniques . 39 2.4.2 Ionisation Cooling . 40 2.4.3 The Cooling Equation . 40 2.5 Multiple Scattering & Energy Loss . 43 2.5.1 The Physical Process . 43 2.5.2 Modelling of Multiple Scattering . 44 2.5.3 Measuring Multiple Scattering: MuScat & MICE . 46 2.5.4 Energy Loss: The Bethe-Bloch Equation and Energy Straggling . 48 2.5.5 Correlations between Scattering and Energy Loss . 50 2.6 Summary . 51 3 The MICE Experiment 52 3.1 MICE Design Goals . 52 3.2 The Beam line . 53 3.2.1 Beam Composition . 54 3.3 Cooling Channel Design . 55 3.3.1 Staged Implementation and Timeline . 55 3.3.2 Co-ordinate System . 56 3.3.3 The Final Channel . 56 3.4 Cooling Channel Components . 57 3.4.1 The Diffuser .
Details
-
File Typepdf
-
Upload Time-
-
Content LanguagesEnglish
-
Upload UserAnonymous/Not logged-in
-
File Pages272 Page
-
File Size-