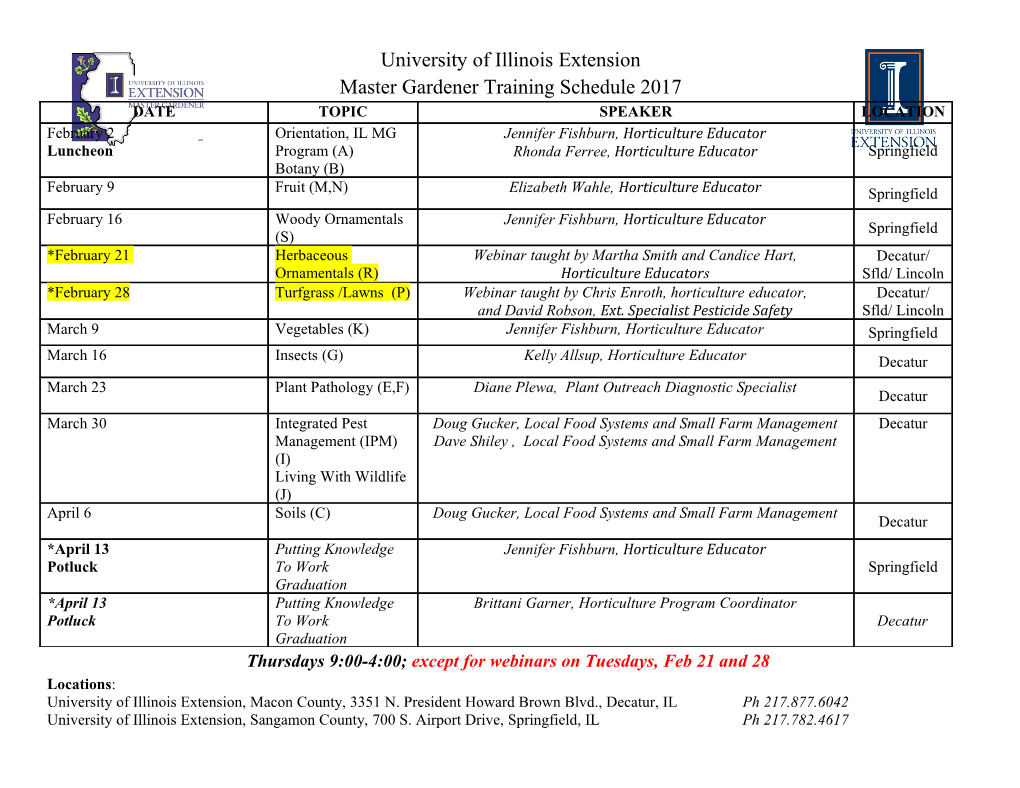
Solar Sails for Planetary Defense & High-Energy Missions Jan Thimo Grundmann, Waldemar Bauer, Kai Borchers, Etienne Dumont, Christian D. Grimm, Tra-Mi Ho, Rico Jahnke, Aaron D. Koch, Caroline Lange, Volker Maiwald, Jan-Gerd Meß, Eugen Mikulz, Dominik Quantius, Siebo Reershemius, Thomas Renger, Kaname Sasaki, Patric Seefeldt, Peter Spietz, Tom Spröwitz, Maciej Sznajder, Norbert Tóth DLR German Aerospace Center, Institute of Space Systems, Robert-Hooke-Strasse 7, 28359 Bremen, Germany [email protected], +49-421-24420-1107 [email protected], [email protected], [email protected], [email protected], [email protected], [email protected], [email protected], [email protected], [email protected], Jan- [email protected], [email protected], [email protected], [email protected], [email protected], [email protected], [email protected], [email protected], [email protected], [email protected], [email protected] Matteo Ceriotti, Colin McInnes, Jens Biele Alessandro Peloni, Christian Krause University of Glasgow DLR German Aerospace Center Glasgow, Scotland G12 8QQ Space Operations and Astronaut United Kingdom Training – MUSC [email protected], 51147 Cologne, Germany [email protected], [email protected] [email protected] [email protected] Bernd Dachwald David Herčík Faculty of Aerospace Engineering Institute for Geophysics and FH Aachen Univ. of Applied Sciences Extraterrestrial Physics Hohenstaufenallee 6 Technical University Braunschweig 52064 Aachen, Germany Braunschweig, Germany [email protected] [email protected] Roy Lichtenheldt, Friederike Wolff Alexander Koncz, Ivanka Pelivan, DLR German Aerospace Center Nicole Schmitz Robotics and Mechatronics Center DLR German Aerospace Center Institute of System Dynamics and Institute of Planetary Research Control Rutherfordstr. 2, 12489 Berlin, Oberpfaffenhofen, Münchener Str. Germany 20, 82234 Weßling, Germany [email protected], [email protected], [email protected], [email protected] [email protected] Ralf Boden, Johannes Riemann, Tobias Mikschl, Sergio Montenegro, Wolfgang Seboldt, Elisabet Wejmo, Michael Ruffer Christian Ziach Informatik 8 Consultants to Universität Würzburg DLR Institute of Space Systems Am Hubland, 97074 Würzburg, [email protected], Germany [email protected], [email protected], [email protected], [email protected] [email protected], wuerzburg.de, [email protected] [email protected] Federico Cordero Simon Tardivel Telespazio-VEGA CNES Darmstadt Future Missions Flight Dynamics Germany 18 avenue E. Belin, [email protected] 31401 Toulouse cedex 9, France [email protected] 978-1-5386-6854-2/19/$31.00 ©2019 IEEE 1 Abstract— 20 years after the successful ground deployment Roadmap NEA Science Working Groups studied small test of a (20 m)² solar sail at DLR Cologne, and in the light of spacecraft concepts. Target-flexible Multiple NEA the upcoming U.S. NEAscout mission, we provide an overview Rendezvous (MNR) [7], Solar Polar Orbiter (SPO) [8], and of the progress made since in our mission and hardware design Displaced L1 (DL1) spaceweather early warning [9] were studies as well as the hardware built in the course of our solar identified as science missions uniquely feasible with solar sail technology development. We outline the most likely and most efficient routes to develop solar sails for useful missions in sail propulsion. Many such studies outline the unique science and applications, based on our developed ‘now-term’ capability of solar sails to provide access to all small solar and near-term hardware as well as the many practical and system bodies (SSSB), at least within the orbit of Jupiter. managerial lessons learned from the DLR-ESTEC GOSSAMER Since, significant progress has been made to improve MNR Roadmap. Mission types directly applicable to planetary trajectory design. [10] [184][185] (cf.[238]) defense include single and Multiple NEA Rendezvous ((M)NR) for precursor, monitoring and follow-up scenarios as well as Although it is relatively easy for solar sails to rendezvous sail-propelled head-on retrograde kinetic impactors (RKI) for with Near-Earth and Potentially Hazardous Objects (NEO, mitigation. Other mission types such as the Displaced L1 (DL1) PHO) in any inclination over the relevant range of semi- space weather advance warning and monitoring or Solar Polar major axis and eccentricity, it remains notoriously difficult Orbiter (SPO) types demonstrate the capability of near-term solar sails to achieve asteroid rendezvous in any kind of orbit, for sailcraft to land on or interact physically with a SSSB from Earth-coorbital to extremely inclined and even target. The German Aerospace Center, DLR, qualified solar retrograde orbits. Some of these mission types such as SPO, sail deployment technologies in the GOSSAMER-1 project. (M)NR and RKI include separable payloads. For one-way [11][13] This technology development continues in the access to the asteroid surface, nanolanders like MASCOT are GOSOLAR large photovoltaic arrays project. [12] an ideal match for solar sails in micro-spacecraft format, i.e. in launch configurations compatible with ESPA and ASAP A Brief History of… secondary payload platforms. Larger landers similar to the JAXA-DLR study of a Jupiter Trojan asteroid lander for the The idea of an outward propulsive force of sunlight, and OKEANOS mission can shuttle from the sail to the asteroids thus of sunlight as a practical source of energy, goes back to visited and enable multiple NEA sample-return missions. The Kepler’s observations on the directionality of comets’ tails high impact velocities and re-try capability achieved by the of 1619. [14] Its magnitude was predicted by Maxwell in RKI mission type on a final orbit identical to the target 1873 [15] and Bartoli in 1876 [16]. That year, the asteroid‘s but retrograde to its motion enables small spacecraft foundations of semiconductor technology were laid by size impactors to carry sufficient kinetic energy for deflection. Adams’s and Day’s discovery of an electrical current driven by selenium exposed to light. [17][18] Kepler’s hypothesis TABLE OF CONTENTS was experimentally demonstrated as pressure due to 1. INTRODUCTION……………………………….2 radiation by Lebedev in 1901 [19] and Nichols and Hull in 1903 [20]. Solar sailing for space propulsion was proposed 2. MOTIVATION....................................................3 first by Oberth [22] and Tsiolkovsky in 1923 and 1924, respectively [21]. Garwin introduced the term ‘solar sailing’ 3. MNR MISSION SCENARIO……………………3 in 1958. [23] It was then considered a key option to go beyond Mars or Venus. Photovoltaics developed from a 4. GOSSAMER-STYLE INTEGRATED LANDERS….4 curiosity [24][175][176] to the power source in space, with 5. PLANETARY DEFENSE EXERCISES…………..6 very few exceptions [25-29]. The discovery of gravity- assists in 1961 made the solar system instantly accessible 6. FUTURE WORK……………………………….6 with available launchers [30]. This disruptive paradigm change from a mostly inaccessible solar system requiring 7. CONCLUSIONS………………………………...6 huge nuclear-electric spaceships [31][32] to the Voyager ACKNOWLEDGMENTS……………………….......6 missions firmly established the combination of chemical propulsion with gravity-assists in solar system exploration REFERENCES…………………………………….7 [33-38] from Earth [39-41]. Fitting space probes into existing fairings advanced electronics and miniaturization. BIOGRAPHY ……………………………………14 [42-44] Nuclear power sources became small niche devices for the outer solar system [45] beyond the extending reach NTRODUCTION 1. I of developed photovoltaics. [177-179] Electric propulsion Any effort which intends to physically interact with only made it into any mission in the 1990s, on photovoltaic asteroids requires understanding of the composition and power. [40][47-50][238] Among the solar sail demonstrators multi-scale surface structure, or even the interior. Mobile launched so far, [51] the sole exception is the Interplanetary Asteroid Surface Scout (MASCOT) landing modules and Kite-craft Accelerated by Radiation Of the Sun (IKAROS) instrument carriers can provide a first access [1-4]. They [52][180], which accompanied JAXA’s atmosphere integrate at the instrument level to their mothership on observation orbiter, AKATSUKI, to Venus. The IKAROS first ‘small’ as well as larger interplanetary missions. [5-6] The demonstrated solar sail effect in space, successfully and as DLR (German Aerospace Center) – ESTEC (European predicted. It also performed the first gravity-assist of a solar th Space Research and Technology Centre) GOSSAMER sail on December 8 , 2010, passing Venus at 80800 km 2 distance and achieving about 20° deflection of theIKAROS’ apply mainly to the launch configuration. Thus, ‘micro‘ trajectory. sailcraft are those which fit the U.S. ESPA or the various Arianespace ASAP and VESPA platforms‘ ‘micro‘ The development of solar sail technology at DLR—A first positions. ‘Mini‘ sailcraft fit the respective larger slots. phase culminated in a successful ground deployment test of [87][88] ‘Nano‘ sailcraft are those small enough to ride in th a (20 m)² boom-supported sail on December 17 , 1999. place of cubesats dispensers, such as NEAscout [81]. Near-term science missions such as ODISSEE and GEOSAIL Together, all these are ‘small‘ sailcraft. however did not materialize. [53-60] Subsequently, the DLR-ESA (European Space Agency)
Details
-
File Typepdf
-
Upload Time-
-
Content LanguagesEnglish
-
Upload UserAnonymous/Not logged-in
-
File Pages21 Page
-
File Size-