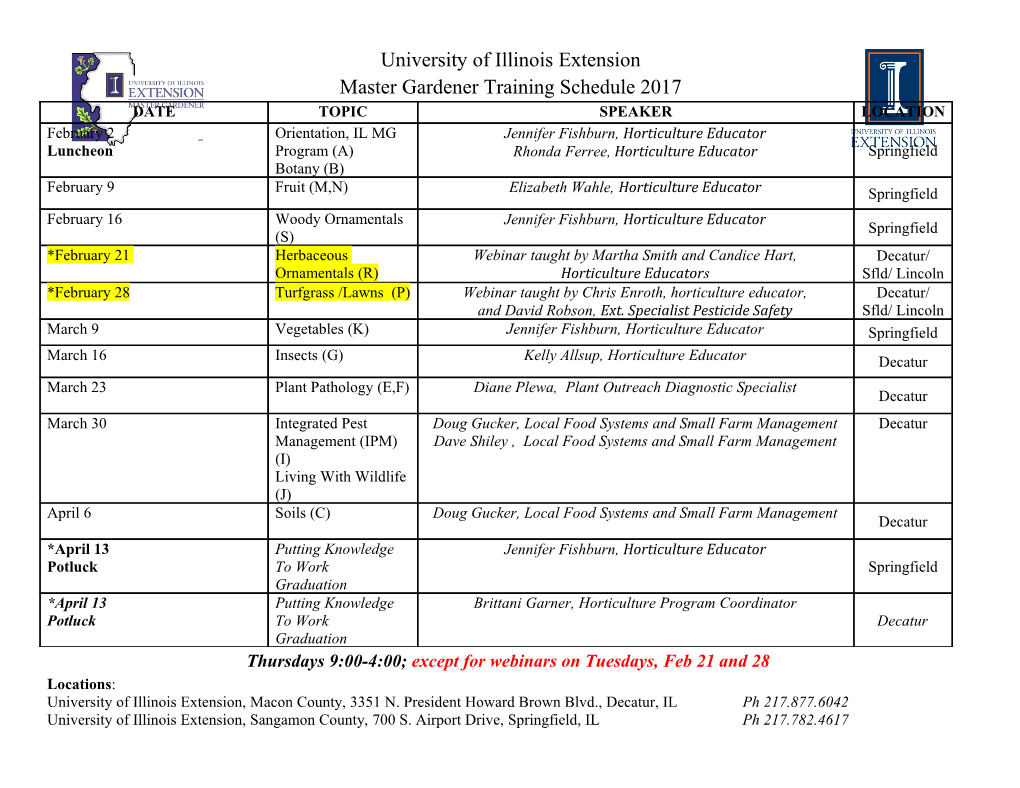
Development of a Method for Measuring Charged Particle Beam Fluence 16 2 Beyond 10 1-MeV-neutron equivalent/cm By Nelly Ayllon Undergraduate Honors Thesis Mentors: Sally Seidel and Martin Hoeferkamp Physics and Astronomy Department University of New Mexico April 22, 2016 1 Abstract A device has been prototyped for measuring remotely and in real time the profile of a charged particle beam and the fluence it delivers to a target. The device was prototyped by building and operating an array of “3D” silicon diodes. 3D silicon sensors are p-n junction-based electronic devices with high tolerance to radiation and durability over time. The motivation for this research is preparation for experiments at the Large Hadron Collider at CERN, an instrument for studying and discovering new particles and forces. This collider will be upgraded in 2024. The upgrade will increase the rate of particle collisions by a factor of 10. Elements of detectors at the collider must be tested in advance to assess their responses to radiation at fluences up to 1x1016 1-MeV-n-eq/cm2. To simulate the damage from particles produced in the collider, the 3D diode array was exposed to a proton beam at Los Alamos National Laboratory. The fluence applied to the sensors was determined by analyzing the leakage current of each sensor as a function of beam exposure time. The potential for imaging the beam profile and measuring its fluence in real time was demonstrated. 2 Contents I. Introduction 1.1 The Large Hadron Collider 1.2 The ATLAS Experiment 1.3 The High Luminosity Large Hadron Collider 1.4 Motivation for the study II. Silicon Sensors 2.1 Description 2.2 Planar versus 3D design 2.3 Measurements of current and capacitance versus bias voltage III. Experiment 3.1 Setup 3.1.1 Temperature readout 3.1.2 Temperature control 3.2 Procedure 3.3 Analysis 3.4 Results 3.4.1 Error analysis 3.4.2 Calibration IV. Recommendations V. Conclusions VI. References VII. Appendix 1: CV and IV plots for all sensors VIII. Appendix 2: Fluence versus beam exposure time 3 I. Introduction A device was developed for measuring remotely and in real time the profile of a charged particle beam and the fluence it delivers to a target. The device was prototyped by building and operating an array of “3D” silicon diodes. The device was tested with an 800 MeV proton beam at the Los Alamos Neutron Science Center (LANSCE). The fluence applied to the sensors was determined by analyzing the leakage current of each sensor as a function of beam exposure time. The potential for imaging the beam profile and measuring its fluence in real time was demonstrated. 1.1 The Large Hadron Collider The Large Hadron Collider (LHC) is part of the European Organization for Nuclear Research (CERN) accelerator complex. It is currently the world’s largest and most powerful particle accelerator. The LHC is used by a worldwide community of over 10000 engineers and scientists from more than 100 countries. The LHC is a 27 km ring of superconducting magnets located 145 m under the French-Swiss Border. There are several structures that serve to accelerate the beam. The devices increase the beam’s particle energy before the collision. The accelerator sends 2 particle beams through different beam pipes. Figure 1 shows the beam pipe at the LHC.The beams are accelerated until they are close to the speed of light. The superconducting magnets guide the beams through the beam pipe. Finally just before the collision quadrupole magnets are used to focus the particles and increase the collision probability. The beams collide at 4 points on the 27 km circumference. The major experiments at the LHC are located at the beam collision areas. The experiments are: ATLAS, CMS, ALICE, and LHCb. Figure 1- Beam pipe at the LHC 145m under the earth’s surface 4 1.2 ATLAS A Toroidal LHC Apparatus or ATLAS is a general purpose detector located at the LHC. The detector was built and is used by a worldwide collaboration involving 3000 people, 174 institutions and 38 countries. The ATLAS experiment seeks answers to fundamental questions about matter, forces and symmetry. The Standard Model is a theory that describes the world. It is a simple and comprehensive theory that explains all the hundreds of particles and complex interactions with only: 6 quarks, 6 leptons, force-transmitters, and the Higgs boson that produces mass. The scientists at ATLAS test the Standard Model predictions and search for new physics processes. The ATLAS detector has dimensions: 46m long, 25m high, and 25m wide. It weighs over 7000 tonnes. The particle beams collide at the center of the ATLAS detector. New particles, products of the collisions, travel in every direction. To detect the particles and record their characteristics, the ATLAS detector has 3 main detector systems: • Inner Detector: For high accuracy measurements of particle trajectories • Calorimeter: Measures the energies of charged and neutral particles • Muon spectrometer: Measures muon paths to determine their momentum Figure 2 shows the ATLAS detector schematically with its main detector systems. The Inner Detector is as close to the beam collisions as 55 mm. The Muon Chambers are 11m away from the collisions[1]. Figure 2-The ATLAS detector, schematic view, showing its main detector systems. 5 1.3 High Luminosity Large Hadron Collider The LHC has been running since 2010, and has produced several scientific discoveries in High Energy Physics (HEP). The LHC started producing proton collisions of 7-8 TeV in 2010. As shown in Figure 3, the LHC schedule includes several upgrades to improve its performance. The LHC will be upgraded to the High Luminosity-LHC (HL-LHC) in 2020.The HL-LHC will increase the collision rate by a factor of 10, allowing researchers to observe rarer events. The collision rate will increase from 1034 cm-2s-1 to 1035 cm-2s-1. The uncertainties will also be reduced due to the higher statistics of the measurements. The upgrade to the HL-LHC presents a challenge. The challenge will not only come from the modifications to the LHC, but also the related upgrade to the ATLAS detector components. The increase in luminosity will produce a significant increase in fluence to the devices installed in the ATLAS detector. The increase in fluence will damage the devices. This damage will decrease the amount of signal detected and increase the noise. The devices require improved radiation hardness characteristics to be able to operate successfully during the remainder at the LHC lifetime. Figure 3- LHC timeline 1.4 Motivation for the study Experimental devices that are going to be exposed to high doses of radiation, such as at the Large Hadron Collider, must be radiation hard. All the devices must be tested in advance to assess their responses to radiation. Particle beams can be used to irradiate devices to high fluences. Imaging the beam profile and measuring its fluence in real time can be a challenge, especially for fluences greater than 1016 1-MeV-neutron equivalent/cm2 [2]. 6 3D silicon sensors are electronic devices with high tolerance to radiation. Previous studies [3,4] that investigated 3D sensors demonstrated their leakage current’s linear response to radiation according to Eq (1): ΔΙ = α Φeq V (1) In Eq (1) Δ� is the change in leakage current, � is a factor that depends in time and temperature, V is the volume of the device, and Φeq is the fluence normalized to that of 1-MeV-neutrons in damage potential. The temperature has a significant effect upon the leakage current recorded for a silicon detector. Eq (2) shows the formula for a scale factor �! 1 1 � �! = exp − − (2). �! �! �!"# If the current is recorded at temperature Ta, it must be scaled to its respective value for temperature Tref by multiplying by � �! . The factor Eg=1.23 eV is the silicon band gap energy and kB is the Boltzmann constant. When the two charged particle beams at the LHC collide, free quarks dominantly form pions in the final state. These pions are the main source of radiation that damages the devices in the detectors. Proton and pion damage are nearely identical for track energies beyond 102 MeV. The typical track energy in the LHC. For that reason it is possible to use a proton beam to simulate pion damage. An 800 MeV proton beam was used to test the device constructed with 3D silicon sensors. The measurements of fluence are given in 1-MeV-neutron equivalent. The damage produced by a hadron is a complicated process that involves the dislocation of atoms from their lattice sites, atomic recoil, ionization, heating, production of complex crystallographic defects, and other things. The conversion between neutrons and proton in terms of their ability to damage the material is characterized by the hardness factor which is 0.71 for 800 MeV protons. 7 II. 3D Silicon sensors 2.1 Description 3D silicon sensors are p-n junction-based electronic devices with high tolerance to radiation and durability over time. The p-type and n-type junctions are micro- machined into the intrinsic Si substrate in columns perpendicular to the substrate surface. The wafer has a resistivity that ranges from 10 to 30 kΩ-cm. To operate, the sensors must have a bias potential applied between their n- and p-type electrodes. The breakdown voltage (Vbd) is found with a measurement of current versus bias voltage (IV). The depletion voltage (Vd) is found with a measurement of bulk capacitance versus bias voltage (CV). The operating voltage is between Vd and Vbd. 3D detectors require low bias voltage to be operated.
Details
-
File Typepdf
-
Upload Time-
-
Content LanguagesEnglish
-
Upload UserAnonymous/Not logged-in
-
File Pages26 Page
-
File Size-