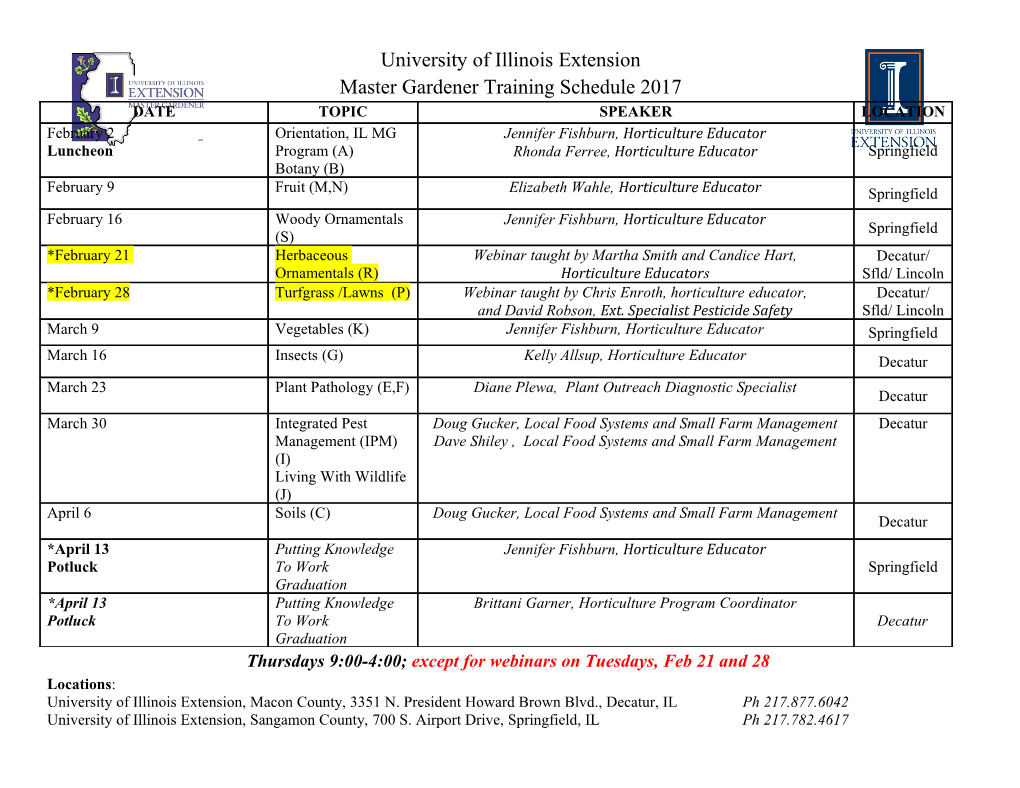
Developmental Neurobiology. edited by Philippe Evrard and Alexandra Minkowski. Nestle Nutrition Workshop Series, Vol. 12. Nestec Ltd., Vevey/Raven Press, Ltd., New York © 1989. Normal Development of Cerebral Neocortex Verne S. Caviness, Jr. Division of Pediatric Neurology, Massachusetts General Hospital and Harvard Medical School, Boston, Massachusetts 02114 The mammalian central nervous system develops through a series of complex cel- lular processes which may be grouped into two broad phases (1,2). The first, cyto- genesis and histogenesis, is a phase in which neurons are formed, move to their correct positions, and elaborate the primary neuritic processes which contribute to emerging dendritic fields and axon fascicles. The second, differentiation and growth, is a phase in which neurons increase in overall size and with respect to the complexity of their structural and molecular organization. During this phase they elaborate terminal dendritic and axonal arborizations and form connections. It is important to realize that the incremental processes, cell generation and growth and synaptogenesis, are not definitive morphogenetic events. The fine de- tails of neuronal structure and organization of circuitry depend upon regressive events which occur terminally during development (3-5). Depending upon the part of the nervous system in question, these include cell death as well as axonal pruning and synaptic elimination. It is probable that these regressive processes serve to opti- mize the final configuration of projections, both quantitatively and with respect to patterns of divergence or convergence. They probably add substantially to the po- tential of the developing nervous system to adapt to injury. Although not emphasized in this chapter, the events of neural development de- pend upon and are closely coordinated with the development of nonneuronal cell populations (6,7). These include the intrinsic neuroglial elements—astrocytes and oligodendroglia. The former play critical structural, supportive, and metabolic roles in the nervous system throughout life. The latter form the myelin investments of ax- ons which are essential to electrical conduction. The cellular events of development unfold through an extended segment of the life of the organism. In man they continue through intrauterine life and well into postnatal life (6-9). The phase of cytogenesis and histogenesis is, relatively speak- ing, quickly accomplished. With respect to the human central nervous system, and in particular the forebrain, the cytogenetic and histogenetic events are largely com- pleted during the first half of gestation. They proceed well into the first postnatal year in the cerebellum. The phase of differentiation and growth proceeds most rap- 1 2 NORMAL DEVELOPMENT OF CEREBRAL NEOCORTEX idly during the second half of gestation and in the first 6 to 12 months of postnatal life. However, it continues at a slower rate much longer. Thus it may continue to shape the structure and adaptive potential of the nervous system of the child through the early school years and even beyond into postpubertal life. It is a corollary of the complexity of developmental events that they are associated with an unrivaled level of risk to the organism (10). In humans the risk of mortality may be as high as 30% during the first trimester of gestation and remains high, as much as eight times that of the general population, through the first six postnatal months. Grossly evident malformation, functionally or cosmetically significant, is characteristic of 3% to 5% of live births, and malformation of the central nervous system is conspicuous among these. The gravest structural anomalies, in general, result from disorders that disrupt cytogenetic or histogenetic events. Among these survival is improbable, and where it occurs, functional disability is grave. Substantial disability may result from disorders of development which do not lead to deformities that are grossly evident at birth. Large numbers of these lesser distur- bances may be ascertained only by their functional consequences, such as distur- bances of learning, language disorders, autism, and recurrent seizures. It is probable that these milder disorders reflect, in most instances, disturbances of the processes of growth and differentiation. CYTOGENESIS AND HISTOGENESIS Neurogenesis The central nervous system is a vast community of neurons, numbering more than 12 billion in man (9,11). The majority of neurons and many glial cells are generated in a specialized pseudostratified columnar epithelium which lines the ventricular cavities. At the ventricular surface, adjacent cells are bound together by specialized intercellular junctions. The subventricular aspect of each cell is free to move to and fro with respect to the plane of fixation at the ventricular surface. During the phase of DNA synthesis, the cell is elongated with the nucleus in an extreme subventricu- lar position. As the DNA cycle enters the G2-phase, the cell constricts to become spherical at the ventricular surface where mitosis occurs. As development proceeds, additional populations of cells undergo their terminal divisions in a subventricular zone, located just distal to the ventricular zone. It is probable that most, perhaps all, cells generated in the subventricular zone are glial. Certain other well-recognized zones of cell generation are located more superficially in the wall of the developing brain. An extensively studied example in the cerebel- lum is the external granular layer, a subpially located generative zone which gives rise to the granule cells, interneurons of the molecular layer, and certain glial spe- cies of the cerebellar cortex. In humans and other primates, a subpial germinal layer (Brunn) arises transiently in the rostrolateral region of the developing cerebrum. The fate of cells arising in this zone is unknown. Additional populations of neurons, NORMAL DEVELOPMENT OF CEREBRAL NEOCORTEX 3 exceptional in their place of origin, include the granular pyramids of the dentate gy- rus of the hippocampal formation and the granule cells of the olfactory bulb. Both these cell classes undergo their terminal divisions within the cortical structures that they inhabit. Their terminal divisions thus occur in positions remote from the periventricular germinal zones which give rise to other neurons of the same cortical structures. Cell Class Determination The neuronal populations of the nervous system are composed of multiple classes, each class distinctive by virtue of its morphology, namely, its general size and patterns of dendritic and axonal arborization (4,9,12). Each class is further dis- tinctive because of its patterns of afferent and efferent connections. Finally, each has a distinctive set of transmitter substances as well as other molecular properties. Within the cerebrum and cerebellum and, perhaps, widely in the central nervous system, determination of cell class is probably completed and irreversible as a con- sequence of molecular-genetic events occurring within the generative zone rather than subsequently in the life history of the cell. The antecedent molecular-genetic events are probably region-specific. Thus, the generative zone of origin of cerebral neocortical neurons gives rise to entirely different neuronal classes than the zone of origin of cerebellar cortical neurons. It is unclear when the molecular events of determination occur with respect to generation order in a given lineage, and this may differ from cell class to cell class. Determination of astrocytic lineage, at least insofar as it is expressed by the synthe- sis of glial fibrillary acidic protein (GFAP), appears to occur with a terminal divi- sion in the ventricular zone (13). With respect to the cerebral neocortex, the principal classes are generated in a set sequence. Possibly each class is an "obliga- tory" product of a terminal division within a given generative sequence (14). Thus cell division in a given sector of the generative zone will form successively the poly- morphic, large pyramidal, stellate, medium and small pyramidal cells, that is, the principal neuronal classes of the neocortex. Factors extrinsic to the dividing cells may have little influence on the determination of class as generation sequences con- tinue. Thus the neuron classes which issue from a region of generative epithelium may in some instances be produced even if cell division proceeds in vitro. This is certainly the case for the granular cells of the cerebellar cortex. Multiple neuronal classes of the cerebral neocortex are able to achieve their distinctive class-character- istic morphologies even when they differentiate in subcortical locations at only a short remove from the ventricular zone (12). It is possible that certain neuronal classes are committed even prior to their termi- nal division. For example, the number of Purkinje cells surviving in the cerebellar cortex of normal mouse-lurcher mutant chimeras is variable but always an integral multiple of 10,000 (15). By extrapolation from the length of the generative cycle and the time of the terminal division, determination of this cell class would occur as 4 NORMAL DEVELOPMENT OF CEREBRAL NEOCORTEX early as the seventh to eighth embryonic day, that is, about the time of neurulation and well before the Purkinje cells undergo their terminal divisions. Cell Deployment The majority of neurons of the central nervous system are generated in epithelia removed, variably, from the positions they will occupy in the adult nervous
Details
-
File Typepdf
-
Upload Time-
-
Content LanguagesEnglish
-
Upload UserAnonymous/Not logged-in
-
File Pages10 Page
-
File Size-