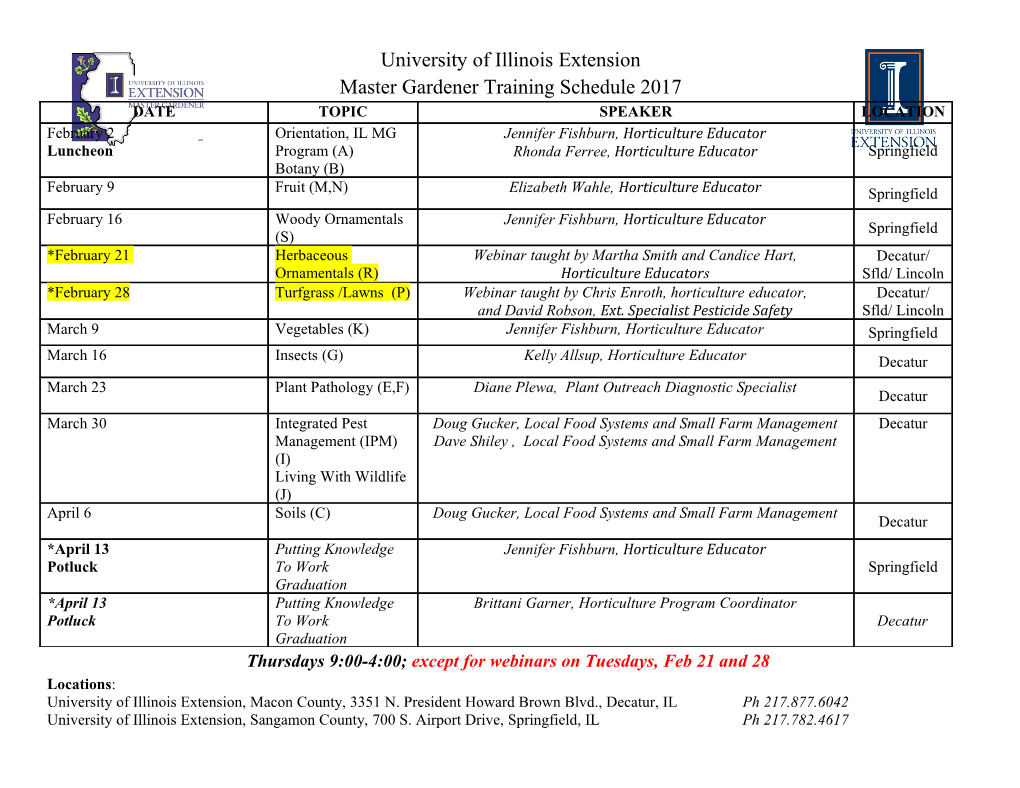
cells Review Intracellular Sources of ROS/H2O2 in Health and Neurodegeneration: Spotlight on Endoplasmic Reticulum Tasuku Konno 1,*, Eduardo Pinho Melo 2 , Joseph E. Chambers 3 and Edward Avezov 1,* 1 Department of Clinical Neurosciences, UK Dementia Research Institute, University of Cambridge, Cambridge CB2 0AH, UK 2 CCMAR—Centro de Ciências do Mar, Campus de Gambelas, Universidade do Algarve, 8005-139 Faro, Portugal; [email protected] 3 Cambridge Institute for Medical Research, University of Cambridge, Cambridge CB2 0XY, UK; [email protected] * Correspondence: [email protected] (T.K.); [email protected] (E.A.) Abstract: Reactive oxygen species (ROS) are produced continuously throughout the cell as products of various redox reactions. Yet these products function as important signal messengers, acting through oxidation of specific target factors. Whilst excess ROS production has the potential to induce oxidative stress, physiological roles of ROS are supported by a spatiotemporal equilibrium between ROS producers and scavengers such as antioxidative enzymes. In the endoplasmic reticulum (ER), hydrogen peroxide (H2O2), a non-radical ROS, is produced through the process of oxidative folding. Utilisation and dysregulation of H2O2, in particular that generated in the ER, affects not only cellular homeostasis but also the longevity of organisms. ROS dysregulation has been implicated in various pathologies including dementia and other neurodegenerative diseases, sanctioning a field of research that strives to better understand cell-intrinsic ROS production. Here we review the organelle-specific ROS-generating and consuming pathways, providing evidence that the ER is a major contributing Citation: Konno, T.; Melo, E.P.; Chambers, J.E.; Avezov, E. source of potentially pathologic ROS. Intracellular Sources of ROS/H2O2 in Health and Neurodegeneration: Keywords: reactive oxygen species; hydrogen peroxide; endoplasmic reticulum; redox signalling; Spotlight on Endoplasmic Reticulum. antioxidants; neurodegeneration; dementia Cells 2021, 10, 233. https://doi.org/ 10.3390/cells10020233 Academic Editor: Gerardo 1. Introduction Z. Lederkremer Substantial quantities of reactive oxygen species (ROS), H2O2 in particular, are pro- Received: 17 November 2020 duced intracellularly. H2O2 is generated endogenously as a functional entity and as a Accepted: 18 January 2021 by-product of reducing/oxidising (redox) chemistry: H2O2 can be generated by regulated Published: 25 January 2021 enzymatic processes to serve as a signal molecule, fuel protein disulfide bonding or as a controlled toxin in immunological-activity. Imperfections in electrochemical reactions Publisher’s Note: MDPI stays neutral can result in unintended H2O2/ROS (e.g., mitochondrial respiration chain with oxygen with regard to jurisdictional claims in as terminal electron acceptor). Some ROS, specifically free radicals, are short-lived as a published maps and institutional affil- product of their high reactivity with biological macromolecules, including nucleic acids iations. and lipids, which in turn can render them hazardous. By contrast, H2O2, a non-radical ROS, is less reactive with biomolecules and as such has a relatively long biological lifespan (cellular half-life of ~1 ms) [1,2]. Yet H2O2 is also capable of producing harmful radicals, through fenton chemistry [3]. Therefore, ROS generating pathways have co-evolved with Copyright: © 2021 by the authors. mechanisms to control their spatio–temporal distribution. Thus, cellular compartments Licensee MDPI, Basel, Switzerland. handling the protein-encoding nucleic acids maintain a strictly reducing environment This article is an open access article (redox potential estimated at −300 mV and −280 mV in the nucleus and cytoplasm, re- distributed under the terms and spectively [4]) (see glossary). These compartments are equipped with ROS quenching conditions of the Creative Commons Attribution (CC BY) license (https:// enzymes, e.g., catalase and glutathione peroxidases. The reducing nature of such envi- creativecommons.org/licenses/by/ ronments allows transient and localised usage of H2O2 as a second messenger. As such, 4.0/). H2O2 complements the cell’s repertoire of second messengers as it can induce rapid and Cells 2021, 10, 233. https://doi.org/10.3390/cells10020233 https://www.mdpi.com/journal/cells Cells 2021, 10, 233 2 of 25 reversible functional changes to proteins through intra/inter molecular disulfide bonding and other cysteine modifications such as sulfenylation and sulfonation. This principle is exemplified by a bacterial H2O2 sensing system, where the key protein, OxyR contains a peroxidatic cysteine residue, surrounded by positive charges, that is preferentially reactive with H2O2. This reaction results in a sulfenic intermediate that is highly reactive with the thiolate of a second “resolving” cysteine, producing an intramolecular disulfide that converts the protein into a functional transcription factor that activates the production of ROS-antagonising enzymes [5]. The kinetic advantage of H2O2, in such a system, over the reducing forces of the cell allows this reaction to prevail even when its concentration is 2–3 orders of magnitudes lower than the antagonising reductants [6]. As such, OxyR has been utilised as a template in engineering a genetically encoded fluorescent H2O2 probe, HyPer, sensitive to physiological H2O2 concentrations [7]. The short-lived nature of H2O2 relative to the biomolecules it reacts with and the overwhelming cellular reductive force ensure the locality and transience of H2O2-mediated signalling events. Several signalling pathways of mammalian cells take advantage of this chemistry, enzymatically producing local H2O2 that can activate factors such as kinases. For instance, oxidation of cysteine residues in SRC, a proto-oncogene non-receptor tyrosine kinase, activates this enzyme, regulating cell proliferation and survival by activating downstream pathways such as ERK and AKT signalling [8]. Several transcription factors, such as NF-κB and KEAP1-NRF2, also sense H2O2 to activate transcriptional programs [9,10]. The reducing, ROS disfavouring environment of the nucleus, cytoplasm and mitochon- dria contrasts with a relatively oxidising milieu in the organelles of the secretory pathway. The endoplasmic reticulum (ER) lumen, in particular, maintains an oxidising environment similar to that of the extracellular conditions. This is favoured by the relatively oxidising reduction potential of glutathione (EGSH/GSSG), a measure of redox conditions, estimated at −208 mV in the ER [11,12]. Moreover, the ER maintains a relatively high turn-over of H2O2 as its oxidative protein folding machinery both produces and utilises H2O2 continuously. H2O2 apparent confinement to the ER (discussed herein) can be compromised when redox homeostasis is perturbed [13,14]. This notion places the ER in the spotlight as a potential source of ROS associated with pathologies, including those associated with neurodegenerative diseases. Excessive ROS load entails damage of structural and functional macromolecules including lipids, protein, RNA, and perhaps with the most severe repercussions to DNA, where a single unrepaired damage event can impact on all its downstream products. As such, compromised insulation of organelles that maintain naturally high ROS (such as ER) may contribute to pathological elevations in ROS. With an emphasis on the ER, here we review the findings contributing to the current understanding of organelles’ ROS producing/consuming pathways and discuss how an imbalance in the activity of ROS generating and antagonising pathways as well as organellar ROS permeability dysregulation can lead to an increase in their steady-state load. The intracellular sources of H2O2 are discussed in the context of their association with neurodegenerative diseases. 2. ER Sources of ROS/H2O2 The ER is devoid of ROS-vulnerable genetic material and therefore, predictably, can tolerate a high abundance of ROS. In addition, H2O2 reactivity with most protein functional groups is energetically unfavourable, suggesting it is unlikely to significantly contribute to protein misfolding directly [15]. ER oxidative protein folding both generates, and is at least partially fuelled by, H2O2 (described in detail below) and basal ER ROS content is reported to be relatively high [13]. This is unsurprising, as approximately 30% of cellular proteins enter the ER, many of them en route to the cell membrane or secretion. A 1970s ex vivo study using rat liver homogenate reports that H2O2 in the microsomal fraction, which contains the ER predominantly, accounts for 45% of the cell total, three times that derived from mitochondrial extracts of the same samples [16,17]. Thus, the ER appears Cells 2021, 10, 233 3 of 25 uniquely capable of producing and utilising H2O2 as fuel for enzymatic oxidative folding process on a massive scale. The core of the ER oxidative folding machinery is a group of oxidoreductase-chaperone enzymes of the protein disulphide isomerase (PDI) family, which functionally interact with their substrates via redox-active CXXC motifs of their catalytic thioredoxin domains (Figure1)[ 18]. PDI itself catalyses disulphide bond formation in substrate proteins, leading to reduction of PDI CXXC motifs that are in turn reoxidised by ER oxidoreductin 1 (ERO1). The later produces H2O2 as two electrons per thiol pair flow from PDIs to molecular oxy- gen [19]. In this process, ERO1 is estimated to consume 25% of the oxygen available in the cell [20–22]. Yet
Details
-
File Typepdf
-
Upload Time-
-
Content LanguagesEnglish
-
Upload UserAnonymous/Not logged-in
-
File Pages25 Page
-
File Size-