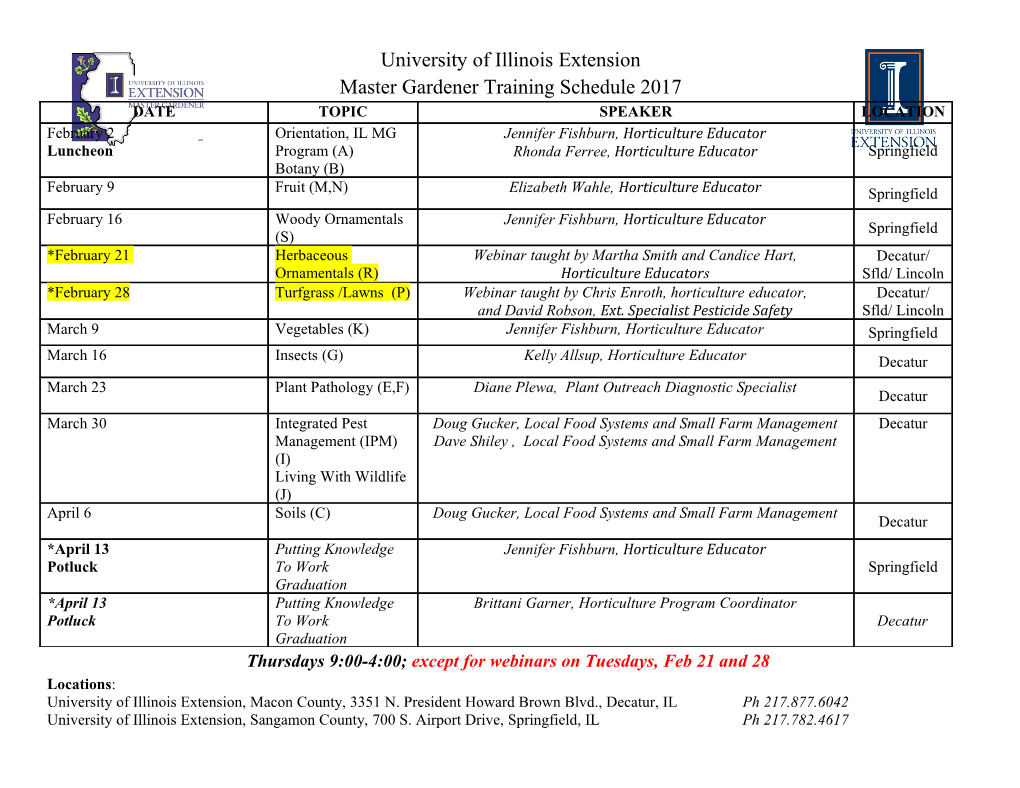
Optical properties of TlNi2Se2 : Observation of pseudogap formation X. B. Wang,1 H. P. Wang,1 Hangdong Wang,2 Minghu Fang,2, 3 and N. L. Wang4,5, ∗ 1Beijing National Laboratory for Condensed Matter Physics, Institute of Physics, Chinese Academy of Sciences, Beijing 100190, China 2Department of Physics, Zhejiang University, Hangzhou 310027, China 3Collaborative Innovation Center of Advanced Microstructures, Nanjing 210093, China 4International Center for Quantum Materials, School of Physics, Peking University, Beijing 100871, China 5Collaborative Innovation Center of Quantum Matter, Beijing, China Quasi-two-dimensional nickel chalcogenides TlNi2Se2 is a newly discovered superconductor. We have per- formed optical spectroscopy study on TlNi2Se2 single crystals over a broad frequency range at various temper- atures. The overall optical reflectance spectra are similar to those observed in its isostructure BaNi2As2. Both the suppression in R(ω) and the peaklike feature in σ1(ω) suggest the progressive formation of a pseudogap feature in the midinfrared range with decreasing temperatures, which might be originated from the dynamic local fluctuation of charge-density-wave (CDW) instability. We propose that the CDW instability in TlNi2Se2 is driven by the saddle points mechanism, due to the existence of van Hove singularity very close to the Fermi energy. PACS numbers: 74.70.Xa,74.70.-b,74.25.Gz,74.72.Kf I. INTRODUCTION measurements14 in this weakly correlated system. More specially, the neutron pair-distribution-function The discovery of iron-based superconductors is the most (PDF) analysis in a combined high-resolution synchrotron x- significant breakthrough in the condensed matter physics in ray diffractionand neutronscattering study in KNi2Se2 reveals recent years1. The iron-pnictides/chacogenides are close to that the local charge density wave (CDW) state is present up to 300 K but disappears on cooling in the ”heavy-fermion” state magnetism and it is widely believed that the spin-fluctuation is 12,13 responsible for the pairing of the superconductingelectrons2,3. below Tcoh . Theeffect is opposite to what is typically ob- served. Moreover the ARPES did not observe any anomaly Compared with the iron-based compounds, the nickel-based 22 systems have much lower critical temperature, for example, in the spectra upon entering the fluctuating CDW state . To 4 5 understand the unique behavior, it is highly desirable to in- LaONiP (Tc = 3K) , LaONiAs (Tc = 2.7K) , BaNi2P2 6 7 vestigate the charge dynamics in the whole temperature range (Tc = 2.4K) , BaNi2As2 (Tc = 0.7K) , usually lower than 5 K even upon doping5. To date, no evidence for ordered or below 300 K. even fluctuated magnetism associated with Ni in proximity to Optical spectroscopy is a powerful bulk sensitive technique superconductivity is found. Moreover, the majority of evi- with high energy resolution, which is widely used to probe dence suggests that the Ni-based systems can be understood the carrier dynamics and possible gap formation of an elec- in context of conventional electron-phonon theory8–11. tronic system. In this work, we present a detailed optical spectroscopy study on TlNi2Se2 single crystals. Our opti- Recently, there nickel chalcogenides KNi2Se2 (Tc ≃ 12 13 cal data reveals that TlNi2Se2 is a good metal with a distinct 0.8K) , KNi2S2 (Tc ≃ 0.46K) , and TlNi2Se2 (Tc ≃ 3.7K)14 were reported, in which superconductivity ap- plasma reflectance edge, similar to its isostructure BaNi2As2. The overall frequency dependent reflectivity R(ω) is gradu- pears to involve heavy-fermion behavior below a coher- −1 15,16 ally suppressed near 2500 cm with decreasing temperature, ent temperature T ∼20 K. In contrast to K Fe − Se , coh x 2 y 2 and a pseudogapfeature appears at any temperaturebelow 300 TlNi2Se2 compound is homogeneous without Ni vacancy or phase separation12,14. Considering the monovalent Tl or K. The weak gap structure is attributed to the local CDW or- K in the stoichiometry composition, the effective valance der or fluctuations. Our measurement indicates that the lo- / of Ni in such compound is ”1.5+”. The mixed valency cal CDW order fluctuations are further enhanced at the lowest ff of Ni1.5+ was proposed to induce the heavy effective band temperature, which is di erent from the neutron PDF analy- arXiv:1507.01690v2 [cond-mat.supr-con] 28 Dec 2015 mass12,13,17. However, very recently, angle-resolved photoe- sis on KNi2Se2 in its ”heavy fermion state”. In Combination mission spectroscopy (ARPES) measurement18 reveals that with the earlier ARPES results, we suggest that the fluctuating 19 CDW instability is driven by the presence of saddle points in TlNi2Se2 shares a universal band structure with BaFe2As2 20 band structure which leads to the van Hove singularity close and BaCo2As2 , with a chemical potential shifted due to 8.5 6 to the Fermi level. more 3d electrons in TlNi2Se2 (3d ) than in BaFe2As2 (3d ) 7 and BaCo2As2 (3d ). Therefore, it can be viewed as heavily 6.5 electron doped TlFe2Se2 (3d ), with reduced electronic cor- 21 II. EXPERIMENT relations than in its cousin KxFe2−ySe2 . Furthermore, the camelback-shaped band at Z point gives rise to a pronounced van Hove singularity (VHS) near Fermi energy (EF), which Single crystals of TlNi2Se2 were grown by the self-flux provides a natural explanation to the heavy electrons behavior method14. Their structure, transport, magnetic and thermody- inferred from the electronic heat and the upper critical field namic properties were already reported14,23. The frequency- 2 dependent reflectance spectra at different temperature were 1.6 300 K ) measured by Bruker IFS 113v and 80v spectrometers in the 200 K -1 −1 100 K ∼ cm frequency range from 30 to 20 000 cm (4 meV 2.5 eV), 1 -1 − ) 1.2 1 ∼ 10 K and then the reflectance was extended to 50 000 cm ( 6.1 -1 eV) at room temperature with a grating-type spectrometer. An ( cm in situ gold and aluminum overcoating technique was used -1 0.8 to obtain the reflectivity R(ω). Considering the small size of 4 0.1 −1 our samples, the data below 100 cm are cut off for reliabil- 100 1000 10000 -1 (cm ) (10 ity. The real part of conductivity σ1(ω) is obtained by the 1 0.4 Kramers-Kronig transformation of R(ω). The Hagen-Rubens TlNi Se relation was used for low frequency extrapolation; at high fre- 2 2 quencyside, an extrapolationmethod using X-ray atomic scat- tering functions was applied to generate the high-frequency 0 3000 6000 9000 12000 24 reflectivity . -1 (cm ) FIG. 2: (Color online). Frequency dependence of the optical conduc- III. RESULTS AND DISCUSSION tivity below 12 000 cm−1 at different temperatures. The inset shows −1 σ1(ω) over broad frequencies up to 50 000 cm on a logarithm scale. Figure 1 shows the reflectance spectra of TlNi2Se2 single crystals over a broad energy scale at various temperatures. The value of R(ω) approaches unity towards zero energy and eral temperature-independent interband transitions could be shows an increase with decreasing temperature, indicating a also well resolved, as shown in the inset of Figure 2. The op- −1 good metallic behavior. By lowering the temperature, we do tical conductivity between 500 and 10 000 cm is gradually not see any sharp changes in the optical spectra but rather suppressed and a broad peak-like feature becomes more and −1 weak suppression of R(ω) near 2500 cm−1 , signaling the more obvious at 2800 cm as the temperature decreases. The 26 opening of a partial gap (pseudogap). With increasing ω, spectral line shape is similar to that of BaFe2As2 across the R(ω) drops quickly near 7000 cm−1 , known as the screened SDW transition though the feature is much weaker. It seems plasma edge. The relativity high edge position reveals a high to suggest that there should also be a density-wave gap open- carrier density, which is similar to isostructural compound, ing in TlNi2Se2 . Furthermore, this pseudogap feature is most 25 BaNi2As2 . There is another edge structure at about 15 000 prominent at low temperature and can be resolved even in the cm−1 which is caused by an interband transition. The reflec- 300 K data. tivity becomes roughly temperature independent at higher fre- To quantify the temperature evolution of optical conductiv- quencies. The inset of Fig. 1 shows R(ω) data up to 50 000 ity, especially the low frequency components, we use a sim- cm−1 in a logarithmic scale at 300 K. ple Drude-Lorentz model to decompose optical conductivity 27 Figure 2 shows the real part of conductivity spectra below spectra : 12 000 cm−1 at different temperatures. The Drude-type con- 2 2 2 ωpi Γ S j Γ jω ductivity is observed for all spectra at low frequency and sev- σ (ω) = Di + (1) 1 X 4π 2 + Γ2 X 4π 2 − 2 2 + 2Γ2 i ω Di j (ω j ω ) ω j 1.0 where ωpi and ΓDi are the plasma frequency and the relax- 0.8 ation rate of each conduction band, while ω j, Γ j and S j are 0.6 the resonance frequency, the damping and the mode strength R 0.8 300 K of each Lorentz oscillator, respectively. This model includes 0.4 Drude and Lorentz terms, which approximately capture the 100 1000 10000 contribution by free carriers and interband transitions. Both TlNi Se -1 2 2 (cm ) 14,23 18 transport and ARPES results suggest the multiband 0.6 R character of TlNi2Se2 , therefore we applied two Drude com- 300 K ponents analysis here, similar to the other members of ”122” 200 K 25–27 100 K system . Even though the Drude-Lorentz fit is somewhat 0.4 10 K arbitrary and the accuracy of the derived fit parameters is un- certain, it will not modify the key outcomes of the analysis.
Details
-
File Typepdf
-
Upload Time-
-
Content LanguagesEnglish
-
Upload UserAnonymous/Not logged-in
-
File Pages5 Page
-
File Size-