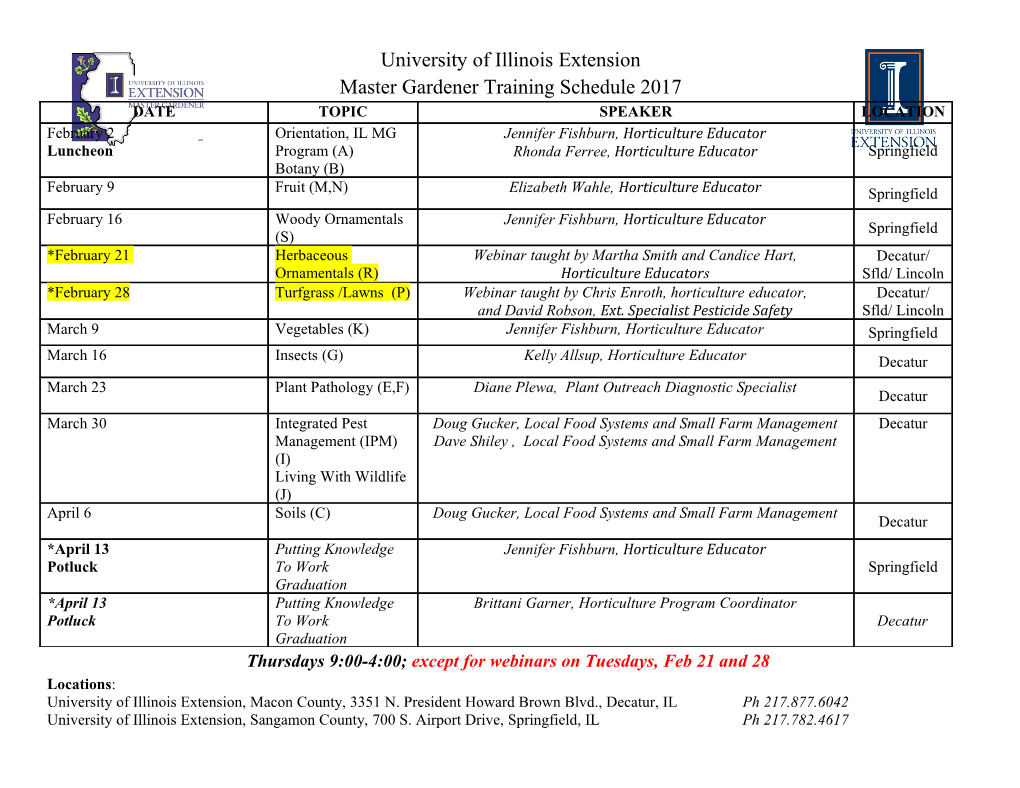
Biogeosciences, 11, 2939–2960, 2014 www.biogeosciences.net/11/2939/2014/ doi:10.5194/bg-11-2939-2014 © Author(s) 2014. CC Attribution 3.0 License. Examination of the role of the microbial loop in regulating lake nutrient stoichiometry and phytoplankton dynamics Y. Li1,2,3, G. Gal4, V. Makler-Pick5,6, A. M. Waite7,*, L. C. Bruce1,7, and M. R. Hipsey1,7 1Aquatic Ecodynamics, School of Earth & Environment, The University of Western Australia, Crawley WA 6009, Australia 2Department of Ecology, Jinan University, Guangzhou 510632, China 3School of Environmental Science & Engineering, Ocean University of China, Qingdao 266100, China. 4Kinneret Limnological Laboratory, Israel Oceanographic and Limnological Research, Migdal 14950, Israel 5Faculty of Civil and Environmental Engineering, Technion-Israel Institute of Technology, Haifa 32000, Israel 6Oranim Academic College of Education, Kiryat Tivon 36006, Israel 7The Oceans Institute, The University of Western Australia, Crawley WA 6009, Australia *current address: Alfred-Wegener-Institut Helmholtz-Zentrum für Polar- und Meeresforschung, Am Handelshafen 12, 27570 Bremerhaven, Germany Correspondence to: M. R. Hipsey ([email protected]) Received: 8 July 2013 – Published in Biogeosciences Discuss.: 16 December 2013 Revised: 3 May 2014 – Accepted: 6 May 2014 – Published: 5 June 2014 Abstract. The recycling of organic material through bacteria cally amplifying internal phosphorus limitation due to bacte- and microzooplankton to higher trophic levels, known as the rial competition for phosphate to satisfy their own stoichio- “microbial loop”, is an important process in aquatic ecosys- metric requirements. Importantly, each configuration led to tems. Here the significance of the microbial loop in influenc- a unique prediction of the overall community composition, ing nutrient supply to phytoplankton has been investigated and we conclude that the microbial loop plays an important in Lake Kinneret (Israel) using a coupled hydrodynamic– role in nutrient recycling by regulating not only the quan- ecosystem model. The model was designed to simulate the tity, but also the stoichiometry of available N and P that is dynamic cycling of carbon, nitrogen and phosphorus through available to primary producers. The results demonstrate how bacteria, phytoplankton and zooplankton functional groups, commonly employed simplifying assumptions about model with each pool having unique C : N : P dynamics. Three mi- structure can lead to large uncertainty in phytoplankton com- crobial loop sub-model configurations were used to isolate munity predictions and highlight the need for aquatic ecosys- mechanisms by which the microbial loop could influence tem models to carefully resolve the variable stoichiometry phytoplankton biomass, considering (i) the role of bacterial dynamics of microbial interactions. mineralisation, (ii) the effect of micrograzer excretion, and (iii) bacterial ability to compete for dissolved inorganic nu- trients. The nutrient flux pathways between the abiotic pools and biotic groups and the patterns of biomass and nutrient 1 Introduction limitation of the different phytoplankton groups were quan- tified for the different model configurations. Considerable One of the principal objectives for water quality manage- variation in phytoplankton biomass and dissolved organic ment of freshwater bodies is to reduce the magnitude and matter demonstrated the sensitivity of predictions to assump- frequency of nuisance algal blooms. Excess nutrients are tions about microbial loop operation and the specific mech- generally implicated in the production of nuisance blooms anisms by which phytoplankton growth was affected. Com- since they fuel primary production and organic matter ac- parison of the simulations identified that the microbial loop cumulation (Elser, 1999). In trying to understand these pro- most significantly altered phytoplankton growth by periodi- cesses much work in limnology is based on the classic “N-P-Z-D” (nutrients-phytoplankton-zooplankton-detritus) Published by Copernicus Publications on behalf of the European Geosciences Union. 2940 Y. Li et al.: Microbial loop effects on lake stoichiometry paradigm, which assumes a relatively simple flow of nutri- organisms and the fluxes between them are in reality neither ents to autotrophic and then heterotrophic pools. However, uniform nor static (Elser and Urabe, 1999; Sterner and Elser, it is now well-documented both in oceanographic and, to a 2002). Whilst representation of microbial loop processes has lesser extent, in limnological applications, that higher order been developed in marine ecosystem models (e.g. Faure et predators such as crustacean zooplankton or fish can be sup- al., 2010), their uptake in freshwater ecosystem models has ported by two paths: the so-called “green” (algal-based) and been limited and none to our knowledge simultaneously re- “brown” (detrital-based) food web components (Moore et al., solve the microbial loop and the dynamic stoichiometry of 2004). The latter refers to the dynamics of the heterotrophic carbon (C), nitrogen (N) and phosphorus (P). bacteria and the microzooplankton grazers (defined here as As a background to this study, there have been several size less than 125 µm that account for rotifers, ciliates and attempts to incorporate the microbial loop into Lake Kin- juvenile macrograzers; Thatcher et al., 1993) – often termed neret ecosystem models. Initially, a steady-state C flux model the “microbial loop”. This has been shown to play an im- was developed to examine C cycling through the plank- portant role in shaping carbon fluxes in lakes and in enhanc- tonic biota, including consideration of the microbial loop ing nutrient cycling at the base of food webs (Gaedke et al., (Stone et al., 1993; Hart et al., 2000). A one-dimensional 2002), including in Lake Kinneret which is the focus in this (1-D) coupled hydrodynamic–ecosystem model (DYRESM- study (Stone et al., 1993; Hart et al., 2000; Hambright et al., CAEDYM) was presented by Bruce et al. (2006), which fo- 2007; Berman et al., 2010). cused specifically on the zooplankton dynamics and their Less well understood is how the microbial loop affects contribution to nutrient recycling. However, the model pre- phytoplankton growth and thus potentially shape patterns of sented by Bruce et al. (2006) had a simplistic representation phytoplankton succession. There are several main mecha- of the microbial loop dynamics, like many contemporary lake nisms by which microbial loop processes are thought to in- ecosystem models, and also did not individually simulate two fluence phytoplankton dynamics: (i) the provision of bacteri- important cyanobacterial species, Microcystis sp. and Aph- ally mineralised nutrients for phytoplankton growth; (ii) the anizomenon sp., which are important to the health of the excretion of readily available nutrients by micrograzers that ecosystem and sensitive to stoichiometric constraints within support primary production (Johannes, 1965; Wang et al., the food web (Zohary, 2004). Gal et al. (2009) expanded this 2009); (iii) the competition of bacteria with phytoplankton model to include a dynamic microbial loop parameterisation for inorganic nutrients when organic detritus becomes nutri- and accounted for the two cyanobacterial species listed above ent depleted (Barsdate et al., 1974; Bratbak and Thingstad, and validated the model approach against a comprehensive 1985; Stone, 1990; Kirchman, 1994; Caron, 1994; Joint et al., data set. The relationship between phytoplankton stoichiom- 2002; Danger et al., 2007). Additionally, a potential fourth etry and patterns in the stoichiometry of available nutrients indirect mechanism is that bacteria provide an alternative was further analysed by Li et al. (2013), who noted that the food source for micrograzers, thus alleviating some grazing microbial loop parameterisation approach could adjust both pressure from small primary producers. The relative signif- the quantity and stoichiometry of nutrient transfers. icance of each of these mechanisms, and in particular how This research builds on these studies and applies the val- they interact in a dynamic environment to shape microbial idated model with the general aim of isolating the signifi- community composition and influence net productivity re- cance of the microbial loop on the phytoplankton patterns mains unclear. within the lake. Specifically, three different microbial loop Models of lake ecosystems are increasingly common to model structural configurations were designed and analysed support management and analysis of water quality prob- to unravel how the microbial loop processes identified above lems, acting as ‘virtual’ laboratories for exploring ecosystem combine to affect (a) the stoichiometry of nutrient trans- processes particularly for questions where empirical studies fers through the planktonic food web, and (b) phytoplank- would be difficult to undertake (Van Nes and Scheffer, 2005; ton growth and community composition. The results high- Mooij et al., 2010). In most models published to date it is light the importance of resolving the variable stoichiometry generally assumed that the biomass of heterotrophic bacteria of microbial interactions in aquatic models and suggest that is fairly stable and that the majority of bacterial production commonly used simplifying assumptions may compromise is lost to respiration (Cole, 1999). As a result, most quan- model function. titative models of carbon and nutrient fluxes in freshwater ecosystems essentially
Details
-
File Typepdf
-
Upload Time-
-
Content LanguagesEnglish
-
Upload UserAnonymous/Not logged-in
-
File Pages22 Page
-
File Size-