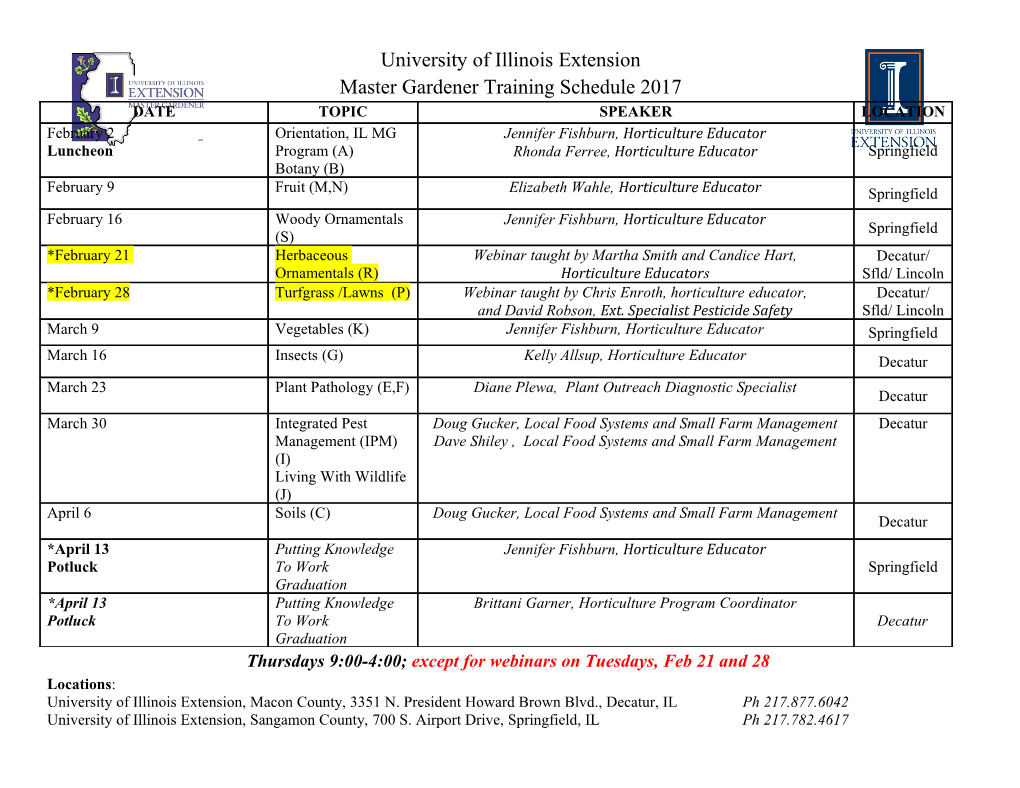
EUROPEAN ORGANIZATION FOR NUCLEAR RESEARCH CERN – AB DEPARTMENT CERN–AB–2004–026 (ABP) CLIC-Note 595 Stabilization of Nanometre-Size Particle Beams in the Final Focus System of the Compact LInear Collider (CLIC) Stefano Redaelli ∗ Abstract The Compact LInear Collider (CLIC) study at the European Organization for Nu- clear Research (CERN) is developing the design of a 3 TeV e+e− linear collider. The discovery reach of this machine depends on obtaining a luminosity of 1035 cm−2s−1, which will be done by colliding beams with transverse spot sizes in the nanometre range ( 60 0:7 nm2). Tolerances on fast mechanical stability of the focusing quadrupo- les≈ reach×the 0.2 nm level. The serious concern of magnet stabilization for future linear colliders has been addressed by building a CERN test stand on magnet stability, bring- ing together state-of-the-art stabilization technology, latest equipment for vibration mea- surements and realistic magnet prototypes. For the first time an accelerator magnet was successfully stabilized to the sub-nanometre level, reducing its vibrations level by one order of magnitude with respect to the supporting ground. The best measurements indi- cate transverse RMS vibration amplitudes (above 4 Hz) of (0.79 0.08) nm horizontally and (0.43 0.04) nm vertically, maintained to a maximum of less than (1.47 0.15) nm and (1.000.10) nm, respectively, over a period of several days. Detailed simulations of time-dependent luminosity, which use a model for magnet displacements based on mea- sured vibration spectra, show that approximately 70% of the CLIC goal luminosity can be achieved with the demonstrated performance in the CERN test stand. This indicates the basic feasibility of colliding nanometre-size beams in CLIC. Geneva, Switzerland 1 June 2004 PhD∗ thesis presented at the University of Lausanne (CH), High Energy Physics Institute (IPHE), in December 2003. Work was carried out at CERN from January 2001 to December 2003, in the framework of the CLIC Stability Study Team. Abstract The Compact LInear Collider (CLIC) study at the European Organization for Nuclear Research (CERN) is developing the design of a 3 TeV e+e− linear collider. The discovery reach of this ma- chine depends on obtaining a luminosity of 1035 cm−2s−1, which will be done by colliding beams with transverse spot sizes in the nanometre range ( 60 0:7 nm2). Transporting the CLIC beams over 15 km, focusing them to nanometre spot sizes≈and colliding× the two separate beams head-on imposes extraordinary requirements on the stability of the magnetic guiding and focusing fields. Tolerances on fast mechanical stability reach the 0.2 nm level. These tolerances have been considered as one of the major obstacles for building high energy e+e− linear colliders. This serious concern has been addressed by building a CERN test stand on magnet stability, bring- ing together state-of-the-art stabilization technology, latest equipment for vibration measurements and realistic magnet prototypes. For the first time an accelerator magnet was successfully stabilized to the sub-nanometre level, reducing its vibrations level by one order of magnitude with respect to the supporting ground. Vibration spectra were recorded over four orders of magnitude. The best measurements indicate transverse RMS vibration amplitudes (above 4 Hz) of (0.79 0.08) nm hori- zontally and (0.43 0.04) nm vertically, maintained to a maximum of less than (1.47 0.15) nm and (1.00 0.10) nm, respecti vely, over a period of several days. The demanding tolerances on mechan- ical vibrations of accelerator magnets are basically achieved. Detailed simulations have been used to predict the achievable luminosity of the collider based on a realistic stability model. This set-up includes a 3D beam transport, a full dynamical 2D model of transverse magnet vibrations (based on experimental data), the measured vibration damping with stabilization technology, a beam-based po- sition feedback and a quantum-electromagnetic model of the beam-beam interaction. The numerical simulation predicts that approximately 70% of the CLIC goal luminosity can be achieved with the demonstrated performance in the CERN test stand. This indicates the basic feasibility of the nanometre-size colliding beams foreseen in the CLIC concept. Further improvements in technology and more advanced fast beam-based feedbacks will allow demonstrating the full design luminosity in the future. Contents 1 Introduction 5 2 The CLIC design and its challenges 6 2.1 Need for a linear collider . 6 2.2 The scheme of a linear collider . 7 2.3 Definition of coordinate system . 8 2.4 The design of the Compact LInear Collider . 9 2.5 Beam delivery systems of linear colliders . 11 2.6 Baseline design of the CLIC beam delivery system . 14 3 Theory and simulations of CLIC luminosity performance 17 3.1 Design luminosity of CLIC . 17 3.1.1 Hourglass effect . 18 3.1.2 Beam-beam effects . 19 3.1.3 Emittance increase from synchrotron radiation . 22 3.1.4 Crossing angle . 23 3.1.5 Simulations of design luminosity for CLIC . 24 3.2 Perturbations of the ideal performance . 27 3.2.1 Luminosity reduction due to beam-beam offsets . 27 3.2.2 Sources of magnet displacements . 31 3.2.3 Tolerances on quadrupole offsets . 34 3.2.4 Frequency analysis of quadrupole offsets . 38 3.3 Counteracting luminosity degradation . 43 3.3.1 Mechanical stabilization of accelerator magnets . 43 3.3.2 Feedback correction of the beam-beam offset . 46 3.3.3 Knobs for spot size tuning . 58 4 Experimental set-up and stabilization techniques 59 4.1 Overview of the laboratory and equipment . 59 4.1.1 The CLIC vibration test stand at CERN . 59 4.1.2 The honeycomb support structure . 61 4.1.3 The CLIC prototype quadrupoles . 61 4.2 Sensor for vibration measurements . 66 4.2.1 Overview of the sensors available at CERN . 66 4.2.2 Seismometric geophones . 68 4.2.3 Capacitive sensors . 75 4.3 Stabilization techniques . 76 4.3.1 Soft air-pressure system . 76 4.3.2 Stiff piezo-based system . 82 5 Measured stability performance of the CLIC quadrupoles 94 5.1 Achieved stabilization of CLIC quadrupoles . 94 5.1.1 Comparison with previously achieved magnet stabilities . 98 5.2 Measured long-term stability . 98 5.2.1 Long-term stability of fast vibration damping . 98 5.2.2 Alignment stability of the support structure . 100 5.3 Low-frequency quadrupole stability . 102 5.4 Water induced quadrupole vibrations . 107 5.5 Transmission of quadrupole supports . 110 5.6 Honeycomb support used as a girder . 113 6 Time-dependent luminosity performance of CLIC 115 6.1 Overview of predicted luminosity performance and assessment of CLIC feasibility . 115 6.2 Effect of uncorrelated quadrupole vibrations . 115 6.2.1 2D model for the time-dependent luminosity simulations . 115 6.2.2 CLIC luminosity with achieved magnet stabilization . 123 6.2.3 Effect of water vibrations on CLIC luminosity . 124 6.2.4 Effect of alignment supports . 125 6.2.5 Comparison of different stabilization technologies . 125 6.3 Effect of slow vibrations . 126 7 Summary 133 8 Acknowledgments 134 9 Bibliography 135 A Assessment of tracking results 145 B Luminosity reduction from offsets and beam size 147 B.1 Luminosity reduction from beam-beam offsets . 147 B.2 Luminosity reduction from beam size variations . 148 C Details of the reference geophones 150 C.1 Measure of sensor resolution . 150 C.2 Frequency calibration . 152 D Comparison of geophones for vibration measurements 154 D.1 Low frequency geophones used at ESRF . 154 D.2 Low frequency geophones used at CERN . 157 E Comparison between geophones and a capacitive sensor 160 E.1 Detail of the capacitive sensor . 160 E.2 Experimental set-up . 161 E.3 Measurement results . 163 F Effect of cooling water on magnet vibrations 167 F.1 Theory of water induced vibrations . 167 F.2 Measurement results . 169 F.2.1 Measurements on the stiff stabilization system . 169 F.2.2 Measurements on the soft stabilization system . 172 4 1 Introduction The discovery reach of a future collider for particle physics depends on its energy and luminosity. Particle physics requirements beyond the Standard Model are demanding and impose many challeng- ing research topics in the technology and design of future accelerators. It has been widely accepted by the international accelerator community (ECFA, ICFA, ACFA, HEPAP) that the next collider beyond the Large Hadron Collider (LHC) should be a high energy e+e− linear collider. Among the various studies, the Compact LInear Collider (CLIC) is the most ambitious with a collision energy of 3 TeV and a target luminosity of 1035 cm−2 s−1, which is approximately 1000 times larger than the lumi- nosity achieved by the Large Electron-Positron Collider (LEP). CLIC is a collaborative study centred at the European Organization for Particle Physics (CERN) with the aim to propose a possible future facility for basic research at the frontier of particle physics. The ambitious goals of the CLIC scheme can only be achieved with significant advances in accelerator technology. Various ongoing studies in- clude the generation of high beam power, the high accelerating gradient in 30 GHz RF structures, the generation of low emittance beams and the stable collision of nanometre-size beams at the interaction point. The feasibility of the CLIC proposal depends on the successful completion of these studies. The stable collision of nanometre-size beams has attracted particular interest over the last years. All linear colliders studies foresee vertical beam sizes at the interaction point from 0.7 nm (CLIC) to 5 nm, in order to achieve the required luminosity with an acceptable electrical power consumption.
Details
-
File Typepdf
-
Upload Time-
-
Content LanguagesEnglish
-
Upload UserAnonymous/Not logged-in
-
File Pages174 Page
-
File Size-