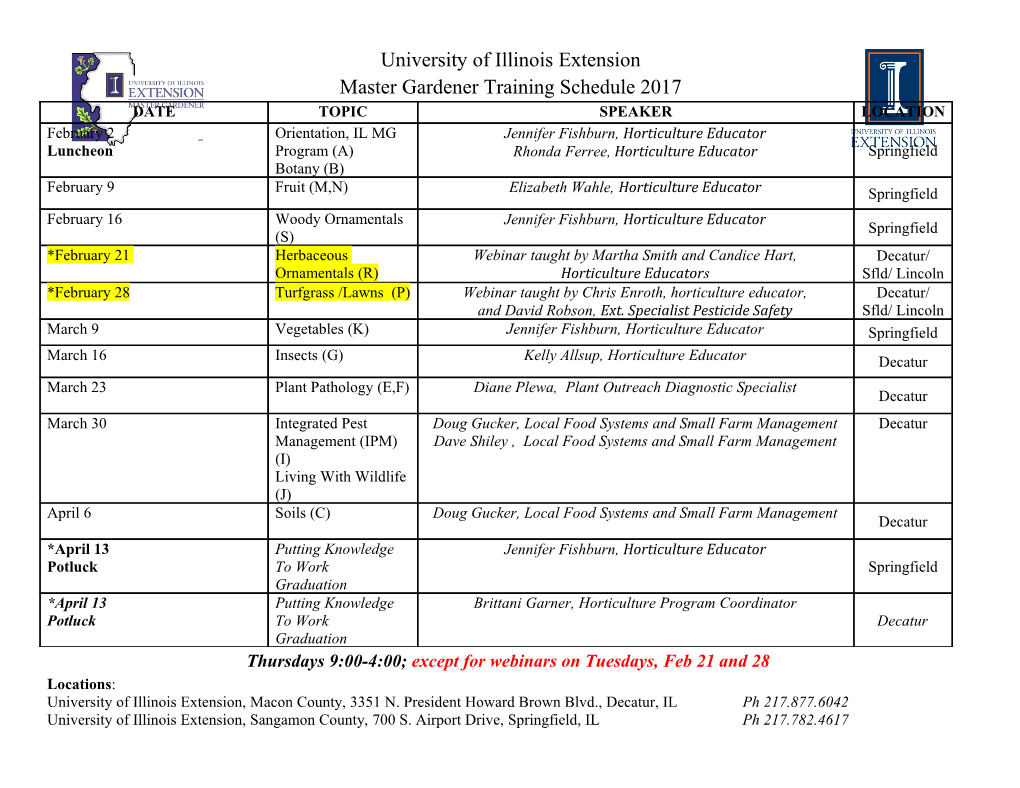
RESEARCH ARTICLE Detrital Thermochronometry Reveals That the 10.1029/2019JF005447 Topography Along the Antarctic Peninsula Key Points: • Major topographic change initiated is Not a Pleistocene Landscape ‐ ~30 12 Ma ago at Bourgeois Fjord, Anna E. Clinger1,2 , Matthew Fox3, Greg Balco2, Kurt Cuffey1,4, and David L. Shuster1,2 Antarctica Peninsula • The timing of topographic change 1Department of Earth and Planetary Science, University of California, Berkeley, CA, USA, 2Berkeley Geochronology was likely in response to the onset of 3 4 alpine glaciation and not in response Center, Berkeley, CA, USA, Department of Earth Sciences, University College London, London, UK, Department of to Plio‐Pleistocene cooling Geography, University of California, Berkeley, CA, USA • Sediment sourcing scenarios scaled by ice velocity cannot completely describe the detrital distribution of Abstract Using offshore detrital apatite (U‐Th)/He thermochronometry and 3D thermo‐kinematic (U‐Th)/He ages modeling of the catchment topography, we constrain the timing of major topographic change at Bourgeois Fjord, Antarctic Peninsula (AP). While many mid‐latitude glacial landscapes developed primarily Supporting Information: fi ‐ • in response to global cooling over the last ~2.6 Ma, we nd that kilometer scale landscape evolution at Supporting Information S1 – • Table S1 Bourgeois Fjord began ~30 12 Ma ago and <2 km of valley incision has occurred since ~16 Ma. This early • Table S2 onset of major topographic change occurred following the initiation of alpine glaciation at this location and prior to the development of a regional polythermal ice sheet inferred from sedimentary evidence offshore of the AP. We hypothesize that topographic change relates to (i) feedbacks between an evolving Correspondence to: topography and glacial erosion processes, (ii) effects of glacial‐interglacial variability, and (iii) the prevalence A. E. Clinger, ‐ [email protected] of subglacial meltwater. The timing and inferred spatial patterns of long term exhumation at Bourgeois Fjord are consistent with a hypothesis that glacial erosion processes were suppressed at the AP during global Plio‐Pleistocene cooling, rather than enhanced. Our study examines the long‐term consequences of Citation: ‐ fi Clinger, A. E., Fox, M., Balco, G., glacial processes on catchment wide erosion as the local climate cooled. Our ndings support the hypothesis Cuffey, K., & Shuster, D. L. (2020). that landscapes at different latitudes had different responses to global cooling. Our results also suggest that Detrital thermochronometry reveals erosion is enhanced along the plateau flanks of Bourgeois Fjord today, which may be due to periglacial that the topography along the Antarctic Peninsula is not a Pleistocene processes or mantling via subglacial till. If regional warming persists and meltwater becomes more landscape. Journal of Geophysical pronounced, we predict that enhanced erosion along the plateau flank will accelerate topographic change. Research: Earth Surface, 125, e2019JF005447. https://doi.org/ 10.1029/2019JF005447 Received 13 NOV 2019 1. Introduction Accepted 27 MAR 2020 ‐ ‐ Accepted article online 14 APR 2020 High relief terrain with overdeepened, U shaped valleys and dissected peaks characterizes many midlati- tude mountain landscapes (e.g., Fiordland, New Zealand; the European Alps; and Coast Ranges of British Columbia) and demonstrates that glaciers play an important role in sculpting alpine topography. Such land- scapes were strongly impacted by Plio‐Pleistocene cooling leading to the onset of alpine glaciations at 2.6 Ma (Muttoni et al., 2003; Raymo, 1994; Tiedemann et al., 1994) and cumulative erosion throughout the subse- quent glacial cycles. Recent work has provided insights about patterns and rates of kilometer‐scale Pleistocene topographic evolution and the question of whether and when the onset of glaciation accelerated erosion in these regions (e.g., Haeuselmann et al., 2007; Shuster et al., 2005, 2011; Valla et al., 2011). Some recent work (e.g., Herman et al., 2013) adopts a broader geographic perspective and tries to assess the global average effect of late Cenozoic glaciation on erosion. Interpreting results from these latter studies and answering the overarching question of “how does glacia- tion induced by cooling affect erosion rates?” require recognizing the diversity of glacial processes in differ- ent climates and that the canonical case of mid‐latitude alpine topography developing in the Pleistocene is only one aspect of the problem. Perspectives from higher‐latitude landscapes, with longer histories of glacia- tion and colder Pleistocene climates, are thus very valuable (e.g., Andersen et al., 2018; Jamieson et al., 2010). A few studies of this sort have demonstrated the importance of the local onset of alpine glaciation (vs. global cooling) as a key control on kilometer‐scale valley incision and that limited erosion occurred during the deep chill of the Pleistocene. For example, glacial processes significantly modified the Patagonian Andes begin- – ©2020. American Geophysical Union. ning by ~10 7.4 Ma (Christeleit et al., 2017; Mercer & Sutter, 1982; Thomson et al., 2010) and subsequently All Rights Reserved. decelerated (Willett et al., 2020). Thermochronometric data from the Lambert Glacier catchment of East CLINGER ET AL. 1of24 Journal of Geophysical Research: Earth Surface 10.1029/2019JF005447 Figure 1. Glacial geomorphology of Antarctic Peninsula. (Left) Bourgeois Fjord, U.S. Navy TMA 2166, frame 296L, 12 January 1969. (Right) Southern end of the Detroit Plateau, U.S. Navy TMA 2143, frame 292L, 12 December 1968. Antarctica indicate (Thomson et al., 2013) that major incision of glacial valleys began ~34 Ma, during the initial expansion of the East Antarctic ice sheet. A major pulse in exhumation was also documented in East Greenland at 30 ± 5 Ma (Bernard et al., 2016), following the initiation of alpine glaciation. A useful framework for interpreting observations of glacial erosion rates from such diverse regions, taken together, and the question of global averaged behavior, is to recognize how progressive climatic cooling transforms the character of glaciation. In most cases, a preglacial landscape will transition first to one of alpine glaciation with temperate and warm‐based (thawed‐bed) glaciers occupying valleys. With further cooling, glaciation expands across the topography, moving warm‐bed regions to lower altitudes and covering highlands with larger regions of frozen‐bed glaciers, which are largely unerosive (e.g., Cuffey et al., 2000). Yet more cooling results in the establishment of polythermal ice sheets and residual regions of alpine glacia- tion with entirely frozen beds and interiors (generically called “polar” conditions) (Cuffey & Paterson, 2010). The timing and completeness of the landscape's transformation along this trajectory have been different in different parts of the globe, providing a range of natural experiments for geomorphological analysis and ren- dering questions of the global averaged behavior of glaciers in specific time periods not particularly mean- ingful on their own. One particular reason to investigate landscapes that have transitioned into the polythermal regime relates to studies of subglacial erosion processes. Accompanying the whole transformation from temperate alpine gla- ciation to polythermal and polar regimes is a reduction in the amount of surface meltwater that reaches the glacier bed. Differences in glacial erosion between polythermal and temperate regimes thus can provide tests of hypotheses about controls on glacier erosion (e.g., Hallet, 1996, 1979; Herman et al., 2011; Koppes et al., 2015); in both regimes, glaciers can move rapidly by basal sliding, but only in the warmer environ- ments does the basal hydrological system transmit large quantities of water that may be essential for sweep- ing the bed free of debris, allowing rapid bedrock erosion, or generating pressure fluctuations that drive quarrying (Alley et al., 2019). In the present paper, we report results from a study of long‐term exhumation of the western Antarctic Peninsula (AP), a landscape of polythermal and polar glaciers that occupies an intermediate position, both geographically and climatologically, between the high polar climate of East Antarctica and the temperate one of Patagonia. The flanks of the AP are a heavily dissected mountainous landscape with 1–2 km of topo- graphic relief and broad overdeepened fjords characteristic of glacial erosion (Figure 1). The first signs of mountain glaciation here appear in offshore sedimentary records as a change in palynomorph assemblages, grain texture, and clay mineralogy at ~37–34 Ma (Anderson et al., 2011), implying a duration of glaciation more than 10 times that for most mid‐latitude landscapes. In addition to the deep fjords and dissected flank valleys, the AP landscape includes a high‐elevation central divide comprising a broad, low‐relief plateau (Figures 1 and 2c), a feature quite unlike the craggy and dissected peaks defining drainage divides in typical mid‐latitude glacial landscapes. This plateau may represent preglacial topography that would have more completely eroded if not for deceleration of erosion caused by cold‐based conditions (e.g., Staiger et al., 2005), which some researchers (Rebesco & Camerlenghi, 2008) hypothesize began at the Plio‐Pleistocene transition. CLINGER ET AL. 2of24 Journal of Geophysical Research: Earth Surface 10.1029/2019JF005447 (a) (b) (c) Figure 2. Modern topography
Details
-
File Typepdf
-
Upload Time-
-
Content LanguagesEnglish
-
Upload UserAnonymous/Not logged-in
-
File Pages24 Page
-
File Size-