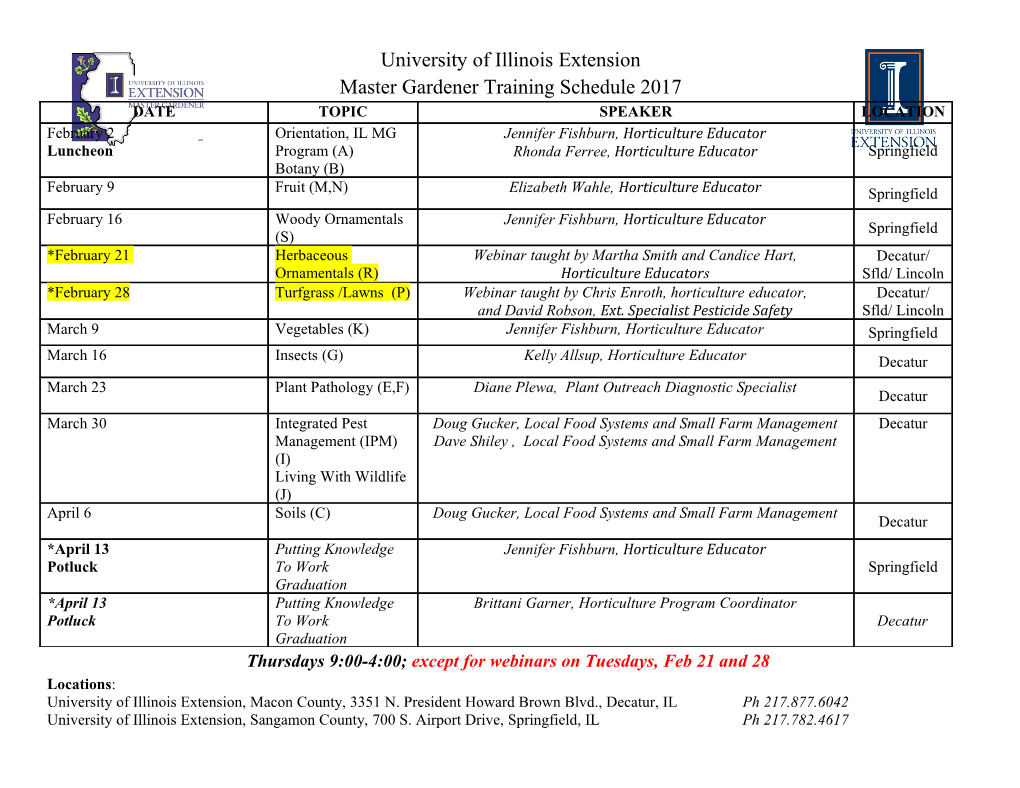
478 MONTHLY WEATHER REVIEW VOLUME 125 The Evolution of the 10±11 June 1985 PRE-STORM Squall Line: Initiation, Development of Rear In¯ow, and Dissipation SCOTT A. BRAUN AND ROBERT A. HOUZE JR. Department of Atmospheric Sciences, University of Washington, Seattle, Washington (Manuscript received 12 December 1995, in ®nal form 12 August 1996) ABSTRACT Mesoscale analysis of surface observations and mesoscale modeling results show that the 10±11 June squall line, contrary to prior studies, did not form entirely ahead of a cold front. The primary environmental features leading to the initiation and organization of the squall line were a low-level trough in the lee of the Rocky Mountains and a midlevel short-wave trough. Three additional mechanisms were active: a southeastward-moving cold front formed the northern part of the line, convection along the edge of cold air from prior convection over Oklahoma and Kansas formed the central part of the line, and convection forced by convective out¯ow near the lee trough axis formed the southern portion of the line. Mesoscale model results show that the large-scale environment signi®cantly in¯uenced the mesoscale cir- culations associated with the squall line. The qualitative distribution of along-line velocities within the squall line is attributed to the larger-scale circulations associated with the lee trough and midlevel baroclinic wave. Ambient rear-to-front (RTF) ¯ow to the rear of the squall line, produced by the squall line's nearly perpendicular orientation to strong westerly ¯ow at upper levels, contributed to the exceptional strength of the rear in¯ow in this storm. The mesoscale model results suggest that the effects of the line ends and the generation of horizontal buoyancy gradients at the back edge of the system combined with this ambient RTF ¯ow to concentrate the strongest convection and back-edge sublimative cooling along the central portion of the line, which then produced a core of maximum rear in¯ow with a horizontal scale of approximately 100±200 km. The formation of the rear-in¯ow core followed the onset of strong sublimative cooling at the back edge of the storm and suggests that the rear in¯ow maximum was signi®cantly in¯uenced by microphysical processes. In a sensitivity test, in which sublimative cooling was turned off midway through the simulation, the core of strong rear in¯ow failed to form and the squall line rapidly weakened. The evolution of the low-level mesoscale to synoptic-scale pressure ®eld contributed to the dissipation of the squall line. Cyclogenesis occurred over Missouri, ahead of the squall line, and caused the presquall ¯ow to veer from southeasterly to southwesterly, which decreased the low-level in¯ow and line-normal vertical wind shear. The reduction in low-level wind shear decreased the effectiveness of the cold pool in sustaining deep convection along the gust front. 1. Introduction scribe that component of the RTF ¯ow near the back edge of the stratiform precipitation region. The strength A common feature of mesoscale convective systems of this rear in¯ow varies substantially from case to case (MCSs) with leading-line/trailing stratiform precipita- (Smull and Houze 1987). Rear in¯ow sometimes com- tion structure is a midlevel jet of rear-to-front (RTF) bines with the internally generated RTF ¯ow to form a storm-relative air¯ow that extends from within the strat- continuous RTF ¯ow across the stratiform precipitation iform precipitation region into the convective region. region. Rear in¯ow is occasionally observed to exceed Occasionally, the RTF ¯ow is con®ned entirely within the strength of the internal RTF ¯ow such that maximum the precipitation regions (Chong et al. 1987), that is, it RTF velocities occur at the back edge of the stratiform is an internal feature of the MCS and is hypothesized precipitation region (e.g., Smull and Houze 1985; Rut- to result from processes internal to the storm (LeMone ledge et al. 1988; Smull and Augustine 1993). 1983; LeMone et al. 1984; Smull and Houze 1987). On The development of the internal RTF ¯ow is asso- other occasions, RTF ¯ow is observed across the back ciated with the formation of a mostly hydrostatically edge of the stratiform precipitation region and was induced midlevel mesolow located to the rear of the termed rear in¯ow by Smull and Houze (1987). In this convective line beneath the sloping convective updrafts; study, we use the term rear in¯ow exclusively to de- alternatively, it can be viewed in terms of the generation of horizontal vorticity by buoyancy gradients along the back edge of the system (LeMone 1983; LeMone et al. Corresponding author address: Dr. Scott A. Braun, National Center 1984; Roux 1985; Fovell and Ogura 1988; Lafore and for Atmospheric Research, P.O. Box 3000, Boulder, CO 80307-3000. Moncrieff 1989; Weisman 1992; Braun and Houze q1997 American Meteorological Society APRIL 1997 BRAUN AND HOUZE 479 1994; Yang and Houze 1995). Several cloud-resolving 1987; Rutledge et al. 1988; Biggerstaff and Houze modeling studies have shown that aspects of the rear 1993). That portion of the RTF ¯ow observed within in¯ow are sensitive to ice microphysical processes the leading portion of the stratiform region and in the (Chen and Cotton 1988; Lafore and Moncrieff 1989; convective region, the internal component of the RTF Szeto and Cho 1994; Yang and Houze 1995). Mesoscale ¯ow, owed its existence primarily to convective-scale modeling studies by Zhang et al. (1989, hereafter ZGP) thermodynamic and dynamic processes, diabatic heat- and Zhang and Gao (1989, hereafter ZG) suggest that ing, and the generation of a mesoscale low-pressure microphysical processes were important in their simu- region beneath the sloping convective updrafts (LeMone lation of a squall line, but that the structure of the rear 1983; LeMone et al. 1984; Smull and Houze 1987; Fov- in¯ow may also have been dependent on the presence ell and Ogura 1988; Lafore and Moncrieff 1989; Weis- of a midlevel mesoscale vortex within the stratiform man 1992; Braun and Houze 1994). This development precipitation region. is not well resolved with the grid spacing of the current Several studies have considered the along-line vari- model (20 km). Consequently, the internal component ability of RTF jets in squall lines. Weisman (1993) dem- of the RTF ¯ow will not be examined. Our analysis onstrated that along-line variability of RTF ¯ow can focuses instead on the mesoscale structure of the rear occur in association with small-scale book-end vortices in¯ow along the back edge of the stratiform precipita- located at the ends of bow echoes with the strongest tion region of the squall line. RTF ¯ow located between the vortices. Skamarock et Microphysical processes played a role in the devel- al. (1994) showed that a similar pattern of RTF ¯ow opment of the rear in¯ow in the 10±11 June squall line occurs on somewhat larger scales with mesoscale vor- (ZG; Gallus and Johnson 1995; Yang and Houze 1995). tices produced at the ends of convective lines. Midlevel ZGP, however, suggested that the location of the max- cyclonic vortices are thought to be particularly impor- imum rear in¯ow near the central portion of the squall tant for cases possessing asymmetric leading-line/trail- line was related to a midlevel mesoscale vortex in the ing stratiform precipitation structure, in which the northern portion of the squall line. ZGP and Zhang strongest convection occurs along the southern portion (1992) showed that this vortex was present in the initial of the line and the stratiform precipitation is concen- environment in association with a midlevel short-wave trated behind the northern portion of the line (Houze et trough and was subsequently enhanced by the convec- al. 1989; Brandes 1990; Houze et al. 1990; Skamarock tive system. The results presented in section 4 suggest et al. 1994; Loehrer and Johnson 1995). Klimowski that the mesoscale structure of the rear in¯ow and the (1994) found that midlevel RTF ¯ow was maximum location of the strongest rear in¯ow along the central near high radar re¯ectivity cores in the convective line, portion of the storm were not the direct result of this which suggests that along-line variability may be partly midlevel mesoscale vortex. Instead, the mesoscale struc- related to the along-line variability of the intensity of ture of the rear in¯ow resulted primarily from a dynamic convection. Circulations associated with the large-scale response to horizontal buoyancy gradients generated in baroclinic environment may also be important in some the rear portion of the squall line. Line-end effects con- cases (ZG). centrated the most intense convection and back-edge While these previous studies have addressed aspects sublimative cooling along the central portion of the of the mechanisms for along-line variability, some ques- storm and these factors led to the development of the tions remain about how the variability of the rear in¯ow most intense rear in¯ow there. This interpretation is is related to storm organization and to microphysical based on an examination of the horizontal variability of processes. Also, are environmental in¯uences important the rear in¯ow relative to the hydrometeor, pressure, for along-line variability of rear in¯ow? and buoyancy ®elds at middle to upper levels. Sensi- This study uses results from a mesoscale model sim- tivity of the mesoscale along-line variability of the rear ulation (described in section 2) of the 10±11 June 1985 in¯ow in the model to resolvable-scale sublimative cool- PRE-STORM1 squall line, which exhibited exception- ing occurred because the large-scale environmental ¯ow ally strong rear in¯ow (Smull and Houze 1987), to in- provided a signi®cant contribution to the strength of the vestigate the three-dimensional structure and evolution rear in¯ow above the 08C level.
Details
-
File Typepdf
-
Upload Time-
-
Content LanguagesEnglish
-
Upload UserAnonymous/Not logged-in
-
File Pages27 Page
-
File Size-